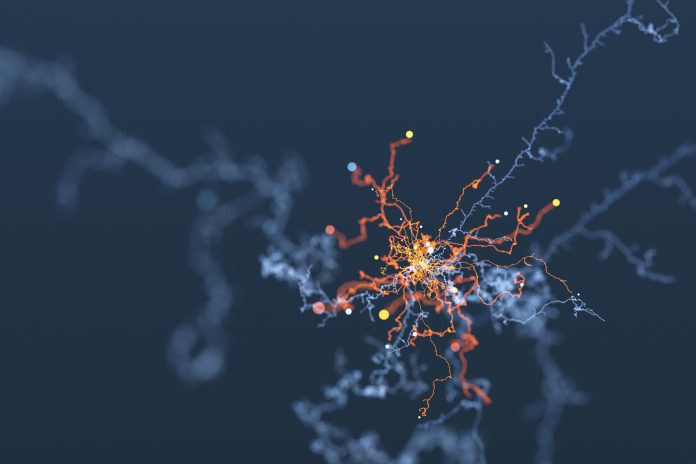
The CorTec Brain Interchange system is a potential tool to improve motor rehabilitation after stroke (Schuettler, 2023). Here, it is sketched how results from studies of other groups are combined to form CorTec’s vision of a new therapy and how first human data was collected to prove the systems therapy capability
In 1949, the neurophysiologist Donald Hebb postulated “Neurons that fire together, wire together,” which became the theoretical fundament for mechanisms underlying motor rehabilitation, e.g., after stroke. Co‐activation of neurons solidifies and even strengthens their synaptic connections. This “learning” can be utilised in conventional, occupational, or physio‐therapeutic rehabilitation approaches. It has been successfully investigated in animal models for decades that electrical stimulation can facilitate this learning process (Bao, 2020). These studies led to the following practical patient studies on stroke rehabilitation (amongst others):
Practical approaches A: Invasive electrical stimulation for stroke treatment
Deep brain stimulation of the cerebellum was applied in combination with conventional rehabilitation in a Phase I study supported by Enspire DBS Therapy, Cleveland, U.S., on 12 patients to treat impairment of the upper extremity. The median improvement was measured to be 7 points in the Upper‐Extremity Fugl‐Meyer Assessment (UEFMA), a scale ranging from 0 (no function) to 66 (no functional deficit). The patients with partial preservation of the distal motor function improved by 15 points UEFMA. (Baker, 2023)
Cortical stimulation was applied in Phase I (8 patients) and Phase II (24 patients) trials, paired with conventional rehabilitation. Clinically meaningful improvements in arm function (Phase I: +10 points UEFMA vs +1.9 in the control group, Phase II: +5.5 points vs +1.9) were achieved (Plow 2014). However, in Phase III (146 patients) supported by Northstar Neuroscience, Seattle, U.S., no significant differences between stimulated patients and control group were found.
Retrospectively, various aspects of the study design are attributed to the surprising negative outcome, including methods of implantation location identification, and considerations on the geometry of peri‐infarct tissue. (Plow, 2009)
Stimulation of the vagus nerve can modulate brain activity, as the vagus nerve fibers project to the brain. In a pilot study funded by MicroTransponder, Austin, U.S., the stimulation was applied in a pivotal study involving 108 patients in combination with conventional rehabilitation to restore upper extremity function. The UEFMA improved in the stimulated patients by 5 points, while the control group improved by 2.4 points (Dawson, 2021).
Practical approaches B: Non-invasive transcranial magnetic brain stimulation (TMS)
TMS utilises electrical coils to push a transient magnetic field into a target tissue, causing eddy currents to be generated in a localised area.
Effectively, this can be considered electrical tissue stimulation without implanted electrodes. It was demonstrated that TMS applied to the brain can facilitate the excitability of neurons, one aspect that contributes to (re‐)learning. This effect can be modulated when applied in a closed‐ loop manner, e.g. when applied in synchrony to brain activity of selected neural networks.
Depending on the selected brain activity rhythm (wave) and the phase angle between the brain wave and stimulus, neural excitability can be up‐ or down‐regulated (Wischnewski, 2022). If up‐regulated, the excitability can be substantially higher compared to an open‐loop TMS (Gharabaghi, 2014).
Lessons learned/conclusion
Electrical stimulation using an implanted device can substantially enhance stroke recovery. Increasing the excitability of brain tissue is most effective when applied in correct synchronicity with brain activity. Combining these two discoveries will unleash new powers in stroke rehabilitation.
First performance tests on humans using the Brain Interchange system
First of all, the system must be capable of continuously recording human brain activity. We anticipate a daily session of up to two hours in combination with physiotherapy.
In a study carried out with the University of Houston, U.S., we demonstrated 24‐hour recording capability in clinical settings by trying to identify spikes in brain signals of epilepsy patients. The results (number of spikes detected) were compared to those of common clinical amplifiers connected to the patient‐implanted electrodes simultaneously. No significant difference in spike detection was observed across the devices (Ayyoubi, 2024).
Furthermore, it was verified with the University of Washington, U.S., that stimulation artefacts do not compromise the system. Electrical stimulation pulses are accompanied by large electrical signals (artefacts), which can be mistaken by the algorithm for a brain wave, causing erratic triggering of further pulses or masking the brain signals of interest. In two of two patients with implanted electrodes, a closed‐loop phased‐dependent stimulation in synchrony to beta activity was successfully conducted (publication in preparation).
Stroke therapy: Outlook
Scientific background, practical clinical studies and first own performance studies with patients of other groups indicate that the Brain Interchange enables a powerful new stroke therapy.
The first step towards the therapy will be starting an early feasibility study later in 2024 with our clinical partners from the University of Washington in Seattle.
References
- Schuettler, Martin. “CorTec’s Brain InterchangeTM system: Revolutionizing brain therapy with closed-loop neuromodulation”, Open Access Government, July, (2023), pp.164-165.
- Bao, Shi-chun, et al. “Rewiring the lesioned brain: electrical stimulation for post-stroke motor restoration.” Journal of Stroke 22.1 (2020): 47.
- Plow, Ela B., et al. “Invasive cortical stimulation to promote recovery of function after stroke: a critical appraisal.” Stroke 40.5 (2009): 1926-1931.
- Plow, Ela B., and Andre Machado. “Invasive neurostimulation in stroke rehabilitation.” Neurotherapeutics 11 (2014): 572-582.
- Baker, Kenneth B., et al. “Cerebellar deep brain stimulation for chronic post-stroke motor rehabilitation: a phase I trial.” Nature Medicine 29.9 (2023): 2366-2374.
- Dawson, Jesse, et al. “Vagus nerve stimulation paired with rehabilitation for upper limb motor function after ischaemic stroke (VNS-REHAB): a randomised, blinded, pivotal, device trial.” The Lancet 397.10284 (2021): 1545-1553.
- Wischnewski, Miles, et al. “The phase of sensorimotor mu and beta oscillations has the opposite effect on corticospinal excitability.” Brain Stimulation 15.5 (2022): 1093-1100.
- Gharabaghi, Alireza, et al. “Coupling brain-machine interfaces with cortical stimulation for brain-state dependent stimulation: enhancing motor cortex excitability for neurorehabilitation.” Frontiers in Human Neuroscience 8 (2014): 122.
- Ayyoubi, Amir Hossein, et al. “Benchmarking signal quality and spatiotemporal distribution of interictal spikes in prolonged human iEEG recordings using CorTec wireless brain interchange.” Scientific Reports 14.1 (2024): 2652.