Archive for category Cognitive Rehabilitation
[ARTICLE] Virtual reality technology in the rehabilitation of post-stroke cognitive impairment: an opinion article on recent findings – Full Text
Posted by Kostas Pantremenos in Cognitive Rehabilitation, Virtual reality rehabilitation on November 9, 2023
Introduction
Post-stroke cognitive impairment (PSCI) is a group of syndromes in which one or more cognitive dysfunctions, such as memory, executive function, orientation and attention, occur within 6 months after stroke (Lim et al., 2021). The prevalence of PSCI is as high as 64%, and 1/3 of these patients will progress to dementia, seriously affecting their quality of life (Delavaran et al., 2017). Post-stroke limb movement disorders have long received widespread attention and have a well-established clinical rehabilitation system (Nakawah and Lai, 2016). However, PSCI is often neglected.
Currently, oral medications and rehabilitation are the main treatment modalities for PSCI, but the therapeutic effects are not satisfactory. Animal models cannot reproduce several features of the pathogenesis of vascular cognitive impairment, which limits the development of relevant drugs (Kuang et al., 2021). To date, no drug for vascular cognitive impairment has been approved by the Food and Drug Administration (USA) (Gorelick et al., 2011). Vascular dementia and Alzheimer’s disease (AD) overlap in their neuropathological mechanisms (Sun, 2018). Drugs for AD can also improve cognitive function and activities of daily living in patients with PSCI, such as donepezil, galantamine and memantine (Glass et al., 2020; Cichon et al., 2021). However, the side effects and potential harms of long-term drug use need to be considered (Shetty, 2013). Cognitive impairment is mainly assessed with paper and pencil tests (De Roeck et al., 2019). The occupational therapist provides therapeutic or adaptive tasks depending on the patient’s cognitive status (Mijajlović et al., 2017). This type of training is tedious and the results vary widely in clinical practice. However, there is no clear treatment for PSCI (Rost and Brodtmann, 2022).
The effective integration of rehabilitation for cognitive impairment with computer technology has led to new and more interesting treatment modalities, such as virtual reality (VR) (Ge et al., 2018). VR is a new computer-generated technology that is being widely used clinically for the diagnosis, assessment, and treatment of mental disorders (Freeman et al., 2017). VR has two key features: immersion and interaction, providing a three-dimensional environment for the user to interact with the virtual environment through multiple sensory channels, including visual, auditory, and tactile (García-Betances et al., 2015). Previous studies have shown that cognitive training, physical training, and lifestyle can improve cognitive function (Stern, 2012; Huang et al., 2017). Compared to traditional rehabilitation therapies, VR incorporates some game-like elements that increase participants’ motivation, cooperation, and satisfaction. VR can be designed with different scenarios and training to target different cognitive areas, and can be adapted in a timely manner according to patients’ needs and cognitive status. In addition, compared to traditional paper-and-pencil tests, VR-based goal-directed navigation and recognition tasks are performed by immersing patients in a 3D virtual environment (La Corte et al., 2019). VR navigation tasks may involve more complex processes, including representational updating, spatial memory, and adaptation of the self when approaching a distant spatial target (Brument et al., 2021). These skills and manipulations cannot be accounted for in paper-and-pencil tests.
VR technology has been initially demonstrated in the treatment of cognitive disorders, such as memory impairment, attention impairment, executive function, language and numeracy impairment (D’Cunha et al., 2019). Several studies have shown that VR technology improves visuospatial motion perception, delayed memory, orientation and attention better than traditional cognitive rehabilitation (Liao et al., 2020; Torpil et al., 2021). Besides, a study by Liao et al. (2019) found significant improvements in self-care and gait in patients who underwent VR interventions (task-based interventions: simulated grocery store, simulated kitchen, finding a store, etc.). A meta-analysis found that VR was less effective in improving cognitive function in patients with AD than in normal elderly and people with MCI. However, the feasibility of VR technology has been demonstrated in a non-immersive VR intervention for patients with AD (Hofmann et al., 2003; Kim et al., 2019). Notably, the effect of the intervention was correlated with patient cooperation. Impaired memory and executive function are key features of AD. Several studies have used VR tasks to intervene with AD patients for 2–4 months (Serino et al., 2017; Oliveira et al., 2021). The results showed that the VR task was effective in improving patients’ overall cognitive function, memory and executive function, especially spatial navigation and memory.
At present, with the rise of virtual reality technology, VR technology has a good prospect of being applied to PSCI. PSCI seriously threatens patients’ quality of life, and VR technology has a potential role in rehabilitation. VR technology applied to the rehabilitation of PSCI not only allows patients to train in a safe and interesting environment, but also provides timely feedback on the effectiveness of treatment. This paper aims to outline the key role of VR technology in improving cognition in patients with PSCI. Moreover, the authors highlight the limitations and challenges of current research related to VR technologies for improving cognition in stroke patients. […]
[ARTICLE] Effects of Cognitive Exercise Therapy on Upper Extremity Sensorimotor Function and Activities of Daily Living in Patients with Chronic Stroke: A Randomized Controlled Trial – Full Text
Posted by Kostas Pantremenos in Cognitive Rehabilitation, Paretic Hand on May 4, 2023
Abstract
This study investigated the effects of cognitive exercise therapy on upper extremity sensorimotor function and daily activity in patients with chronic stroke and compared these effects to those of conventional occupational therapy. The 30 patients with chronic stroke (mean age: 63.6 ± 12.7 years; height: 162.8 ± 8.1 cm; weight: 60.6 ± 7.6 kg; body mass index: 22.8 ± 1.9 kg/m2) were divided into two treatment groups with 15 patients in each. The respective interventions were provided for 30 min per day, five times weekly for 4 weeks. Manual and sensory function tests were conducted to evaluate the sensorimotor function, while the Korean-Modified Barthel Index was used to assess daily activities. All outcome variables were assessed before and after the interventions. A significant interaction was observed in sensory function (p = 0.001) but not motor function or daily activities (p > 0.05). No significant main group effects were found for any outcome variables (p > 0.05). The experimental group showed significant improvements in motor function (p < 0.001), sensory function (p < 0.001), and daily life activities (p = 0.001) after cognitive exercise therapy, whereas the control group showed significant improvement only in daily life activities post-intervention (p = 0.012). These results demonstrated the positive effects of cognitive exercise therapy on upper extremity sensorimotor function and daily life activities and the lack of improvement in motor and sensory function following conventional occupational therapy in patients with chronic stroke. Thus, the combination of cognitive exercise and conventional occupational therapies may be an effective way to improve sensory function and upper extremity motor function in patients with chronic stroke.
1. Introduction
A stroke, a sudden impairment of body function caused by a blockage of blood flow to the brain, has the third-highest mortality rate after cancer and heart disease [1,2]. More than 85% of patients with stroke experience hemiplegia, and 55–75% of these patients have upper extremity impairment [3]. Impaired upper extremity function may result in decreased mobility of the shoulder joint, muscle weakness, sensory impairment, spasticity, and lack of coordination [4]. Subsequently, these lead to sequelae such as limited joint movement and limited upper extremity function [5]. Sensory impairment occurs in at least 50% of stroke patients and is expected to be higher with accurate examinations [6]. Sensory impairment interferes with correct movement and sharply reduces movement based on sensory input or feedback [7]. Patients with sensory impairment avoid movement, and their movements are dull and uncoordinated [7,8]. Thus, for patients with sensory impairment, proprioception, tactile sensation, pressure sensation, and stereoscopic sensation are essential for smooth movement by activating natural movements [9]. As these sensations are closely related to functional recovery after stroke, the impaired sensory function is one of the most significant factors hindering rehabilitation in patients with stroke. Additionally, impairment of upper extremity and sensory functions reduce the performance of basic daily activities. Therefore, patients cannot perform these activities independently and rely on the help of their caregivers [10].
Many interventions have been applied to address upper extremity function in patients with stroke. Among these, task-oriented upper extremity training improved the function of affected upper extremities [11,12,13]. The complex interaction between the left and right brains during bilateral tasks of the upper extremities enhanced the function of the affected upper extremity [11,12]. Moreover, constraint-induced movement therapy in the affected upper extremity of patients with stroke improved upper extremity function [13]. However, these interventions are therapeutically accessible only to patients with at least minimal voluntary motor skills. Another intervention is mirror therapy, which reorganizes brain areas along with physical rehabilitation. Based on the theoretical mirror neuron system, this intervention method promotes functional recovery of the upper extremities by inducing the recovery of motor function and movement on the affected side [14]. Another invention is mental practice, which allows patients to acquire and improve motor skills through thoughts of certain movements rather than actual body movements [15]. When a certain level of sensory recovery can be expected, repeated stimulation may provide necessary sensory feedback; moreover, the senses may be improved to a conscious level by focusing on a given sense [16].
Cognitive exercise therapy is a therapeutic intervention that aims to reorganize the central nervous system through learning motor function recovery [17,18]. This therapy emphasizes the close relationship of motor function to the activation of cognitive processes in the brain, such as perception, attention, memory, judgment, and language. The quality of recovery depends on the correct identification of the cognitive factors [19]. Cognitive exercise therapy allows various movements or actions to be performed through cognitive training processes, rather than movement training through interaction between the body and environment, to build a brain schema with four principles. The first principle is ‘attention’, which focuses on enhancing the effectiveness of treatment and reorganizing exercises in the treatment process. Second, patient treatment requires attention to somatosensory information with the eyes closed. Third, specific treatment instruments or tools are used to treat cognitive problems through interactions between the body and the environment. Finally, cognitive exercise does not force patients to conduct muscle contractions to accurately mobilize motor units. As such, the purpose of cognitive exercise therapy is not to teach patient-specific body postures but rather to develop and maximize the ability to organize the spatial, temporal, and intensity factors of the exercise sequence in the interaction between the body and environment [20].
Studies on cognitive exercise therapy in patients with stroke have shown improved upper extremity functions such as motor function, manual skills, and strength through image training of cognitive exercise therapy [21]. A comparison of cognitive exercise profiles showed improvements in sensory recognition and movement in the paralyzed arm [22]. In another study, activities of daily living (ADL) and motor function of the upper extremity improved after cognitive exercise therapy through contact tasks using a sponge on the paralyzed arm and spatial tasks using a graphic panel in patients with acute stroke. In addition, cognitive exercise profiling improved joint angle, spatiality, and shape recognition in recognition patterns [23].
However, most studies on cognitive exercise therapy were single-case or single-group studies with non-randomized groups and without a control group. Thus, these effects have not been directly compared. In addition, while various assessments have been conducted on upper extremity functions and daily activities, changes in cognitive patterns and pathological characteristics were described rather than used to directly assess sensory function recovery, which is a key factor in cognitive exercise therapy. While this approach is suitable for a single case study or qualitative research with a small number of subjects, it is difficult to quantify the objective results. Therefore, this study evaluated the effects of cognitive exercise therapy and compared them to conventional occupational therapy on upper extremity motor function, sensory function, and daily activities in patients with chronic stroke. We hypothesized that cognitive exercise therapy would improve upper extremity motor and sensory function and daily activities compared to conventional occupational therapy.[…]

(A) Shoulder joint recognition training by motor imagery. (B) Shoulder and elbow joint recognition training using a circular track plate. (C) Training on awareness of elbow and wrist joint angles using a Bogen. (D) Training on pressure awareness of the elbow and wrist using a sponge. (E) Finger tactile recognition training using a tactile plate.
[ARTICLE] Neurocognitive, physiological, and biophysical effects of transcranial alternating current stimulation: Trends in Cognitive Sciences
Posted by Kostas Pantremenos in Cognitive Rehabilitation, REHABILITATION on March 13, 2023
Highlights
- Transcranial alternating current stimulation (tACS) is a non-invasive method for brain stimulation. It is the application of alternating currents to the scalp that pass through head tissues and create an electric field in the brain, which modulates neural activity.
- The induced oscillatory electric fields can bias neural spike timing, synaptic plasticity, and long-range coherence.
- Neurophysiological effects can translate into changes in brain oscillatory power, frequency, and phase connectivity. Thereby, tACS can modulate cognitive and behavioral processes.
- tACS-induced brain modulation enables therapeutic applications in neurological and psychiatric conditions.
- Personalizing stimulation parameters such as intensity, waveform, and location can improve tACS efficacy. Further, advances in higher-dose interventions, multi-electrode network stimulation, and adaptive closed-loop applications show promise for future research.
Abstract
Transcranial alternating current stimulation (tACS) can modulate human neural activity and behavior. Accordingly, tACS has vast potential for cognitive research and brain disorder therapies. The stimulation generates oscillating electric fields in the brain that can bias neural spike timing, causing changes in local neural oscillatory power and cross-frequency and cross-area coherence. tACS affects cognitive performance by modulating underlying single or nested brain rhythms, local or distal synchronization, and metabolic activity. Clinically, stimulation tailored to abnormal neural oscillations shows promising results in alleviating psychiatric and neurological symptoms. We summarize the findings of tACS mechanisms, its use for cognitive applications, and novel developments for personalized stimulation.[…]

[WEB] Exercise Just Once a Month Can Boost Later Cognitive Function
Posted by Kostas Pantremenos in Cognitive Rehabilitation on March 9, 2023
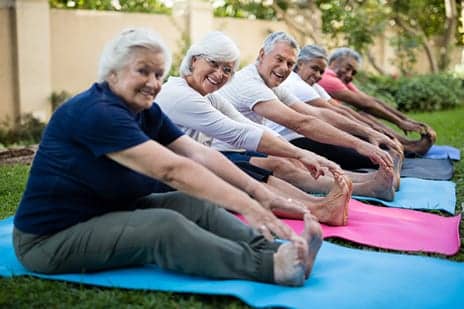
A STUDY FOUND EXERCISING AS INFREQUENTLY AS ONCE A MONTH WAS LINKED TO IMPROVED COGNITIVE FUNCTION LATER IN LIFE.
A new study led by UCL researchers has found that exercising at least once a month at any time in adulthood is linked to better cognitive functioning in later life.
The study, published in the Journal of Neurology, Neurosurgery, and Psychiatry, looked at data from 1,417 people who completed surveys about their leisure-time physical activity (sports and exercise) over three decades and took cognitive tests at the age of 69.
The research team found that people who reported being physically active at least one to four times a month in five separate surveys, aged 36, 43, 53, 60-64, and 69, had the most significant cognitive effect.
This effect was more significant than for those who reported frequently exercising (more than five times a month) during at least one survey period but who did not necessarily keep this up across multiple surveys.
“Our study suggests that engaging in any leisure-time physical activity, at any point in adult life, has a positive effect on cognition,” said Sarah-Naomi James, PhD, lead author. “This seems to be the case even at light levels of activity, between once to four times a month. What’s more, people who have never been active before, and then start to be active in their 60s, also appear to have better cognitive function than those who were never active.”
The study participants were from the 1946 British Birth Cohort, the UK’s longest-running population-based cohort who were all born in the same week in 1946 and whose health has been tracked throughout their life.
The researchers aimed to investigate if there was a period of life when physical activity was essential for later-life cognitive function, in the same way that cardiovascular health in middle age appears to be more important for later cognitive health than during other times of life.
But, rather than finding that one period of life was more important than others, they concluded that starting some form of physical activity and maintaining it over a long time may be more important than the timing of this activity.
At age 69, study participants took several cognitive tests, including the Addenbrooke’s Cognitive Examination, which assesses overall cognitive state and is used to screen people for cognitive impairment. They also did a word learning test and a visual processing speed test, where they were asked to cross out all instances of a particular letter in a page of text.
The researchers also looked at other factors that might explain the link between exercise and cognitive functioning.
They found that, while a link remained after accounting for many other factors, including mental health and cardiovascular health, half to two-thirds of the association could be explained by education level, childhood cognition, and socioeconomic background.
People who engaged more in physical activity were also more likely to have taken A-levels and gone to university, had parents from a more privileged background, and done better in tests at the age of eight, and these factors may separately contribute to better cognitive function in later life.
However, a separate link remained between exercise and cognitive function, and the researchers said more work was needed to understand this link better.
The research was supported by Alzheimer’s Research UK (ARUK) and the Medical Research Council and involved researchers from the MRC Unit for Lifelong Health & Ageing at UCL and the UCL Queen Square Institute of Neurology.
[BLOG POST] Robot-assisted neurocognitive rehabilitation of the hand
Posted by Kostas Pantremenos in Cognitive Rehabilitation, Paretic Hand, Rehabilitation robotics on February 12, 2023
Authors: Irina Benedek, Oana Vanta
1 Innovations in neurorehabilitation of the upper limbs
2 A multimodal approach to robot-assisted neurocognitive rehabilitation of the hand
4 The roles of robotic devices in improving upper extremity function
5 Results, discussions, and future perspectives
6 Conclusion on robot-assisted neurocognitive rehabilitation of the hand
Innovations in neurorehabilitation of the upper limbs
Is robot-assisted neurocognitive rehabilitation of the hand effective? Stroke is the leading cause of mortality and disability worldwide, negatively impacting the overall quality of life of patients. Most stroke survivors suffer from different types of motor impairments, despite the progressions in the field. Since neurorehabilitation of the upper limbs remains challenging, robotic-assisted therapies have been studied and developed in recent years. These methods were established as safe and practical therapies were used additionally to neurorehabilitation programs [1]. More precisely, in the last two decades, robotic devices that focused on training the proximal upper extremity were assessed, showing results similar to dose-matched conventional therapies [2].
For more information on upper limb neurorehabilitation, visit:
- The feasibility of repetitive sensory stimulation in the rehabilitation of the upper limb after a stroke (the PULSE-I study)
- Wearable elbow robot in rehabilitation after a stroke
- Recovery of precise hand movements after stroke
A multimodal approach to robot-assisted neurocognitive rehabilitation of the hand
Distal arm sensorimotor function is essential for improving the quality of life after a stroke. Nevertheless, the distal arm function is crucial for the ability to perform daily activities and is usually severely affected after a cerebrovascular accident when there is a low probability of regaining its full function [3]. However, scientific data demonstrates the possibility of recovery with intensive motor training provided by robotic-assisted devices [4, 5]. Until now, the focus has been on movement practice without implementing a therapeutic paradigm adapted to the capabilities of the technology in question.
In the study by Ranzani et al. [6] from 2020, the goal was to investigate sensorimotor robotic-assisted rehabilitation of hand function and cognition training in patients with subacute stroke. The researchers based their comprehensive approach on the fact that cognition is essential for appropriate interactions between body and environment, such as [6]:
Figure 1. Importance of Cognition
Secondary objectives derived from the hypothesis are that neurocognitive robotic-assisted hand recovery would also improve motor, sensory, and cognitive functions in this category of patients. This method is particularly relevant for hand rehabilitation due to the importance of the cognitive processing of sensory information. Furthermore, combining multimodal inputs requires the involvement of associative cortices that are essential for learning and, consequently, neuronal plasticity and recovery [7]. Even though few studies have compared neurocognitive therapies to other rehabilitation treatments [8, 9], preliminary evidence suggested promising results in the following:
- Enhancing upper limb function
- Improving the ability to conduct daily tasks
- The overall quality of life [8,9].
Inspired by the neurocognitive approach, the authors [6] implemented this concept on a robotic-assisted device focused on exercises including grasping and pronation-supination. Virtual objects were produced by the robot, both visually and haptically, simulating the tangible materials used in traditional recovery [6].
Study design
The study conducted by Ranzani et al. [6] was a randomized control trial that took place in Switzerland. Patients were randomly assigned into two groups: the robot-assisted group (RG), which benefited from neurocognitive therapy with “ReHapticKnob” (Fig. 2), and the control group (CG), which received dose-matched classical neurocognitive therapy. With the main focus on hand function, all participants had three neurocognitive therapy sessions per day over four weeks. Each session lasted 45 minutes, and in the RG, one session was replaced by robot-assisted therapy. The subjects were under the careful supervision of a specialized therapist who adapted the intensity and difficulty level of the exercises according to every patient. The type and number of exercises performed in the RG group were also assessed to match the therapy type and dose performed in conventional recovery programs [6].
Figure 2. Picture of a subject with stroke, using the rehabilitation robot ( available from [6])Between April 2013 and March 2017, 33 patients who suffered a subacute stroke were evaluated and included in the study. Seventeen subjects were randomized in the RG, while 16 were allocated to the CG. 27 patients benefited from the allocated therapy and completed the T1 assessment, while 23 completed the entire protocol. Six patients withdrew before the T1 evaluation due to medical reasons or lack of motivation. No adverse events related to the interventions were noted [6]. The eligibility criteria are described in Figure 3 below:
Figure 3. Patient Eligibility
The roles of robotic devices in improving upper extremity function
As mentioned above, the neurocognitive method proposed in this study implied both sensorimotor and cognitive components, which are equally important during the performance of complicated tasks and daily activities. Patients were instructed to explore different objects, such as sponges, sticks, and strings, to discern their properties by using haptic and postural senses. A robotic device is an ideal therapeutic instrument for performing such tasks because it can render a complex range of stimuli in a repeatable, well-controlled manner [10].
The neurorehabilitation program provided in the RG group consisted of seven types of exercises [6], as showcased in Figure 4 below:
Figure 4. Types of Exercises
The motor aspects of the intervention included symmetric thumb and finger flexion/extension and forearm pronation/supination, executed separately or combined. The sensory aspects included encoding the following types of somatosensory signals without visual information: sponge/spring stiffness, object shape and size, arm positioning, and vibratory cues. The cognitive element of the training, executed passively (with guidance from the robot or therapist) or actively (by the subject), required the elaboration/recognition of perceptual information (object length, stiffness) and encoding/decoding of this information in working memory for comparison purposes of more than one item, along with planning and executing the specific motor plans [6].
The study’s primary outcome was evaluating the changes in upper limb extremity motor impairment from baseline to the end of treatment (T0-T1). The parameter used to quantify the results was the Fugl-Meyer Assessment of the Upper Limb Extremity scale (FMA-UE). Different motor, sensory and cognitive scales were also used for the secondary outcomes. The average number of task repetitions and therapy intensity were assessed to compare the two study groups in terms of dose matching. For the acceptance of the neurocognitive robotic-assisted program, a 4-item questionnaire was developed. The two study groups were evaluated and compared for all outcome measures after the intervention (T-T0) and at the regular follow-ups (T2-T0 and T3-T0) [6].
Results, discussions, and future perspectives
According to the equivalence analysis, the change in FMA-UE in the robotic-assisted group may be regarded as non-inferior to the control group. Both groups showed improvements in all secondary clinical scores after 4 weeks of therapy (T1). In addition to motor impairments, sensory and cognitive deficits were also improved in both groups after 4 weeks of treatment (T1) [6]. However, by the end of the study, subjects randomized in the RG group tended to show better results. At T2 and T3, the changes in FMA-UE were also sustained. When comparing changes in clinical measures over time, the two groups did not demonstrate any significant between-group differences [6].
According to the supervising therapist, the RG did an average of 71.49 task repetitions during a treatment session, compared to 73.47 in the Control Group. There was no statistically significant difference in therapy intensity between the two groups when comparing robot-assisted and traditional therapy sessions [6].
Regarding neurocognitive therapy sessions, the average daily quantity of occupational therapy and/or lower limb kinesiotherapy did not differ statistically between the groups.
The acceptance of the neurocognitive robot-assisted therapy was evaluated by using a self-reported questionnaire answered by 12 patients [6], as showcased in Figure 5:
Figure 5. Patient responses
Unlike other robot-assisted rehabilitation studies that focus primarily on locomotor training, this method fully utilized the robot’s haptic rendering capabilities and suggested a therapy regimen tailored to this potential [6].
Ranzani and the team were able to demonstrate that this strategy was well-liked and recommended by the majority of patients. It could also be included in the patient’s daily routines in the subacute stage of stroke recovery. Although the questionnaire pointed out slight pain in finger fixation and difficulty levels were sometimes evaluated as excessively high in three out of seven exercises [6], most subjects considered the program encouraging and pleasant, and they noticed tangible benefits to their health after completing it.
The findings of the equivalency test assessing the evolution in the FMA-UE show that motor recovery in the RG was not inferior to the CG for the specific intervention. Usually, little to no difference between traditional and robotic-assisted therapies should be expected since the dosage and therapeutic movements are meant to be identical between the study groups. Furthermore, the idea that a traditional treatment session might be replaced without compromising the overall rehab programs opens up new possibilities for robot-assisted therapy development [6].
Conclusion on robot-assisted neurocognitive rehabilitation of the hand
This research reported the findings of a randomized controlled trial that aimed to compare robotic-assisted recovery to conventional therapy for patients with a subacute stroke and impairment of hand function. Although the study included a limited number of participants, the results showed that robot-assisted treatments might be successfully incorporated into clinical neurorehabilitation routines [6]. In other words, this trial is also an indicator of how low data can become significant data. Compared to conventional dose-matched neurocognitive programs, the results demonstrated that this innovative approach was at least as practical as the conventional one.
Early exposure of stroke patients to using such patient-tailored robot-assisted therapy programs opens the door to using such technology in the clinic with little therapist supervision or at home after hospital release to help enhance the dose of hand therapy for stroke patients [6].
Bibliography
- Veerbeek JM, Langbroek-Amersfoort AC, van Wegen EE, Meskers CG, Kwakkel G. Effects of robot-assisted therapy for the upper limb after stroke: a systematic review and meta-analysis. Neurorehabil Neural Repair. 2017; 31(2):107–21. doi: 10.1177/1545968316666957
- Maciejasz P, Eschweiler J, Gerlach-Hahn K, Jansen-Troy A, Leonhardt S. A survey on robotic devices for upper limb rehabilitation. J Neuroeng Rehabil. 2014;11(1):3. DOI: 10.1186/1743-0003-11-3
- Fischer HC, Stubblefield K, Kline T, Luo X, Kenyon RV, Kamper DG. Hand rehabilitation following stroke: a pilot study of assisted finger extension training in a virtual environment. Top Stroke Rehabil. 2007;14(1):1–12. DOI: 10.1310/tsr1401-1
- Lambercy O, Dovat L, Yun H, Wee SK et al. Effects of a robot-assisted training of grasp and pronation/supination in chronic stroke: a pilot study. J Neuroeng Rehabil. 2011;8(1):63. doi: 10.1186/1743-0003-8-63.
- Hsieh YW, Lin KC, Wu CY, Shih TY, Li MW, Chen CL. Comparison of proximal versus distal upper-limb robotic rehabilitation on motor performance after stroke: a cluster controlled trial. Sci Rep. 2018;8(1):2091. doi: 10.1038/s41598-018-20330-3
- Ranzani R, Lambercy O, Metzger JC, Califfi A et al. Neurocognitive robot-assisted rehabilitation of hand function: a randomized control trial on motor recovery in subacute stroke. J Neuroeng Rehabil. 2020 Aug 24;17(1):115. doi: 10.1186/s12984-020-00746-7.
- Van de Winckel A, Wenderoth N, De Weerdt W, Sunaert S et al. Frontoparietal involvement in passively guided shape and length discrimination: a comparison between subcortical stroke patients and healthy controls. Exp Brain Res Springer. 2012;220(2):179–89. doi: 10.1007/s00221-012-3128-2
- Sallés L, Martín-Casas P, Gironès X, Durà MJ et al. A neurocognitive approach for recovering upper extremity movement following subacute stroke: a randomized controlled pilot study. J Phys Ther Sci. 2017;29(4):665–72. doi: 10.1589/jpts.29.665
- Lee S, Bae S, Jeon D, Kim KY. The effects of cognitive exercise therapy on chronic stroke patients’ upper limb functions, activities of daily living and quality of life. J Phys Ther Sci. 2015;27(9):2787–91. doi: 10.1589/jpts.27.2787
- Metzger J-C, Lambercy O, Califfi A, Dinacci D et al. Assessment-driven selection and adaptation of exercise difficulty in robotassisted therapy: a pilot study with a hand rehabilitation robot. J Neuroeng Rehabil. 2014;11(1):154. Available at: https://jneuroengrehab.biomedcentral.com/articles/10.1186/1743-0003-11-154
[BLOG POST] Decision-Making After Brain Injury: Improving Executive Skills
Posted by Kostas Pantremenos in Cognitive Rehabilitation, TBI on January 20, 2023
Medically reviewed by Elizabeth Denslow, OTR/L — written by Flint Rehab.
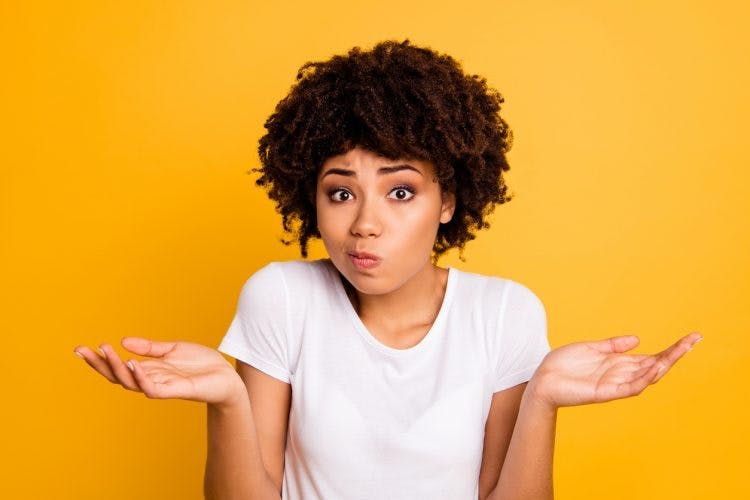
The ability to make efficient and rational decisions is an essential part of everyday life. When this function is impaired, it can make it difficult to perform daily tasks and live independently. Fortunately, survivors can improve their decision-making after brain injury with a proper rehabilitation plan and support from loved ones.
This article will discuss the causes of impaired decision-making after brain injury, and the most effective ways to boost recovery and maximize your independence.
Use the links below to jump straight to any section:
- How a TBI affects decision-making
- Contributing factors of impaired decision-making
- Can decision-making skills improve after TBI?
- Techniques to help you make decisions after TBI
How A TBI Affects Decision-Making
Executive function refers to a variety of cognitive skills that help us to learn new information and manage daily activities such as planning, organizing, and multitasking. Executive function also includes high-level skills such as self-awareness, understanding social cues, and making decisions with good judgment.
All executive function skills are housed in the frontal lobe, primarily within the foremost section referred to as the prefrontal cortex. Therefore, damage to the frontal lobe or prefrontal cortex after a brain injury can impair an individual’s ability to execute these skills, referred to as executive dysfunction. When executive function is impaired, individuals often have difficulties with making and carrying out decisions.
Researchers identified several specific sections of the frontal lobe that play a crucial role in decision-making, including:
- Dorsolateral Prefrontal & Anterior Cingulate Cortex: are associated with cognitive control skills, including response inhibition, conflict monitoring, and switching. These regions of the frontal lobe help with the ability to think before taking action or acting on certain impulses.
- Orbitofrontal, Ventromedial, & Frontopolar Cortex: are associated with value-based decision-making, which can include logical or rational thinking. These sections of the frontal lobe allow you the ability to choose between two appealing options.
When these regions of the frontal lobe sustain damage, it can severely impact the ability to make decisions, among other cognitive functions. However, decision-making is a complex process that involves many other areas of the brain, including the hippocampus (responsible for memory) and Wernicke’s area (responsible for language comprehension).
Contributing Factors of Impaired Decision-Making After Brain Injury
There are many contributing factors that can result in executive dysfunction and impair decision-making after brain injury, including:
- Amnesia: the inability to remember information, caused by damage to the hippocampus. This can impact decision-making as both new information and existing memories are reflected upon to make an appropriate decision.
- Receptive aphasia: caused by damage to Wernicke’s area, this condition involves difficulty with comprehending spoken language, among other language challenges. This can create problems with understanding verbal questions, such as “Would you like to go to the park, library, or store?”, subsequently making it impossible to make a decision.
- Adynamia: a condition involving weakness and a lack of motivation after brain injury, which can interfere with decision-making skills. Adynamia may seem like laziness or depression but is often a result of frontal lobe damage. It can decrease motivation and the desire to initiate or complete an activity. Individuals with adynamia may struggle with motivation to make and execute decisions.
- Attention Deficits: may occur as a result of a frontal lobe brain injury. Attention problems are a common secondary effect that can make it challenging to complete tasks, maintain conversations, and make decisions. Some individuals may have difficulty focusing long enough to think clearly and make a rational decision. Others may be able to make a decision, but may lose focus before being able to actually execute the decision.
- Anosognosia: a condition that involves a lack of insight after brain injury, which can also affect decision-making after a frontal lobe injury. The ability to recognize, understand, and reflect on a situation is important to be able to make rational decisions. Lacking the awareness of oneself or others can lead to poor judgments and hasty decisions.
To address the root cause of impaired decision-making after brain injury, it’s important to consult with your doctor and obtain a proper diagnosis. With a proper diagnosis, you can embark on a rehabilitation regimen that can help you improve executive function skills.
Can Decision-Making Skills Improve After TBI?
Yes, it is possible to improve your decision-making skills after brain injury. After a TBI, many of the neural pathways necessary to execute functions may be damaged or destroyed. However, the brain has the ability to heal and rewire itself through neuroplasticity.
Neuroplasticity helps strengthen existing neural pathways and create new ones. With stronger neural connections, the brain is able to execute functions properly, including decision-making.
To stimulate the brain and activate neuroplasticity, you must engage in high repetitions of cognitive exercises. Practicing a skill consistently helps the brain recognize that skill and strengthen its neural pathways. Therefore, massed practice is crucial in order to stimulate neuroplasticity as much as possible and maximize your chances of restoring cognitive function.
One of the best ways to get started with high repetition of cognitive exercises is to work with an occupational therapist or speech-language pathologist (SLP). Both professionals can provide you with cognitive exercises to improve your decision-making and other executive function skills. They can also make detailed assessments of your ability level and provide you with a more suitable rehabilitation plan.
However, to further maximize your chances of recovery, it’s important to maintain a proper exercise regime at home. Exercising in between your therapy sessions is crucial to stimulate the brain and keep neuroplasticity activated. To boost your motivation, you can use the CT Speech and Cognitive Therapy App.
Designed by two SLPs, the CT App provides access to over 100,000+ cognitive exercises that you can practice from anywhere with a smartphone or tablet. It starts by assessing your current ability level and then assigns exercises tailored to your goals. The CT App is a great way to achieve high repetition of cognitive exercises and improve your decision-making skills after brain injury.
Techniques to Help You Make Decisions After TBI
Though improving your decision-making skills can be challenging, there is hope for recovery. In addition to cognitive exercises, there are other techniques that can help you make rational and clear decisions after a brain injury.
This can include a combination of the following:
1. Address the Root Cause
Impaired decision-making can be caused by a variety of factors including difficulties with language comprehension, adynamia, or attention deficits. To improve these skills, it’s important to work with a therapist and address the root cause first. For example, you may discover that your memory was affected by a TBI, and is interfering with your ability to retain information and make decisions. In this case, improving your short-term memory skills first is necessary in order to improve decision-making skills.
2. Narrow Down Your Options
After addressing the root cause, you may begin to see improvements and start making decisions on your own. When this happens, it can be easy to feel overwhelmed by all the choices you have to make, especially with important decisions. To help reduce feelings of stress and becoming overwhelmed, it can help to narrow down your options. Having fewer options, such as 2-3, can help you manage tasks better and combat feelings of uncertainty. If you are able, try to narrow down the options on your own. However, it may also be helpful to request that your close friends and family provide a couple of options for you to choose from, rather than leaving questions open-ended.
3. Set Time Limits
Some individuals may dwell on the various options they are presented with, however big or small. When this occurs, it helps to set limits that allow you an opportunity to make a decision in a timely manner. For instance, if you are placing an order, set a timer on your phone. When it goes off, order what you feel sounds best. This can encourage you to make quick and decisive decisions.
4. Slow Down
For some individuals, it can be tempting to act impulsively and make inappropriate decisions. To help combat impulsive decision-making after brain injury, it helps to create different habits. For example, before agreeing to a decision, you can try waiting for at least five minutes, and think of the pros and cons. Take your time and do not rush, even if others are waiting for you to make a decision. If it is an especially important decision, consider writing down your options and rationale before making a final choice.
5. Seek Advice
Lastly, as you improve your decision-making skills, be sure to seek advice from trusted friends and family members. Making decisions after a brain injury can be challenging, and the weight of the decision (such as how important or life-changing it is) may add to the challenge.
To help lower the pressure of making a decision, ask a loved one to help you analyze it. They can help you make a pros and cons list or give you their honest opinion. Sometimes seeing things from another perspective can help lighten the load and ease your mind.
Following these techniques can help you make appropriate, rational decisions in a timely manner. A neuropsychologist may also be able to provide you with more personalized solutions to help you improve your decision-making after brain injury.
Improving Decision-Making After Brain Injury
Impaired decision-making after brain injury can make it challenging to navigate everyday life and make the appropriate choices. Fortunately, executive function skills can be improved by having a consistent rehabilitation plan.
We hope this article helped you understand how a brain injury can affect decision-making skills, and the best ways to maximize your recovery.
[Abstract] INCOG 2.0 Guidelines for Cognitive Rehabilitation Following Traumatic Brain Injury, Part III: Executive Functions
Posted by Kostas Pantremenos in Cognitive Rehabilitation, TBI on January 8, 2023
Abstract
Introduction: Moderate-to-severe traumatic brain injury (MS-TBI) causes debilitating and enduring impairments of executive functioning and self-awareness, which clinicians often find challenging to address. Here, we provide an update to the INCOG 2014 guidelines for the clinical management of these impairments.
Methods: An expert panel of clinicians/researchers (known as INCOG) reviewed evidence published from 2014 and developed updated recommendations for the management of executive functioning and self-awareness post-MS-TBI, as well as a decision-making algorithm, and an audit tool for review of clinical practice.
Results: A total of 8 recommendations are provided regarding executive functioning and self-awareness. Since INCOG 2014, 4 new recommendations were made and 4 were modified and updated from previous recommendations. Six recommendations are based on level A evidence, and 2 are based on level C. Recommendations retained from the previous guidelines and updated, where new evidence was available, focus on enhancement of self-awareness (eg, feedback to increase self-monitoring; training with video-feedback), meta-cognitive strategy instruction (eg, goal management training), enhancement of reasoning skills, and group-based treatments. New recommendations addressing music therapy, virtual therapy, telerehabilitation-delivered metacognitive strategies, and caution regarding other group-based telerehabilitation (due to a lack of evidence) have been made.
Conclusions: Effective management of impairments in executive functioning can increase the success and well-being of individuals with MS-TBI in their day-to-day lives. These guidelines provide management recommendations based on the latest evidence, with support for their implementation, and encourage researchers to explore and validate additional factors such as predictors of treatment response.
Similar articles
- INCOG 2.0 Guidelines for Cognitive Rehabilitation Following Traumatic Brain Injury: Methods, Overview, and Principles.Bayley MT, Janzen S, Harnett A, Teasell R, Patsakos E, Marshall S, Bragge P, Velikonja D, Kua A, Douglas J, Togher L, Ponsford J, McIntyre A.J Head Trauma Rehabil. 2023 Jan-Feb 01;38(1):7-23. doi: 10.1097/HTR.0000000000000838.PMID: 36594856
- INCOG 2.0 Guidelines for Cognitive Rehabilitation Following Traumatic Brain Injury, Part IV: Cognitive-Communication and Social Cognition Disorders.Togher L, Douglas J, Turkstra LS, Welch-West P, Janzen S, Harnett A, Kennedy M, Kua A, Patsakos E, Ponsford J, Teasell R, Bayley MT, Wiseman-Hakes C.J Head Trauma Rehabil. 2023 Jan-Feb 01;38(1):65-82. doi: 10.1097/HTR.0000000000000835.PMID: 36594860
- INCOG recommendations for management of cognition following traumatic brain injury, part III: executive function and self-awareness.Tate R, Kennedy M, Ponsford J, Douglas J, Velikonja D, Bayley M, Stergiou-Kita M.J Head Trauma Rehabil. 2014 Jul-Aug;29(4):338-52. doi: 10.1097/HTR.0000000000000068.PMID: 24984096
- INCOG 2.0 Guidelines for Cognitive Rehabilitation Following Traumatic Brain Injury, Part I: Posttraumatic Amnesia.Ponsford J, Trevena-Peters J, Janzen S, Harnett A, Marshall S, Patsakos E, Kua A, McIntyre A, Teasell R, Wiseman-Hakes C, Velikonja D, Bayley MT, McKay A; INCOG Expert Panel.J Head Trauma Rehabil. 2023 Jan-Feb 01;38(1):24-37. doi: 10.1097/HTR.0000000000000840.PMID: 36594857 Review.
- Technological aids for the rehabilitation of memory and executive functioning in children and adolescents with acquired brain injury.Linden M, Hawley C, Blackwood B, Evans J, Anderson V, O’Rourke C.Cochrane Database Syst Rev. 2016 Jul 1;7(7):CD011020. doi: 10.1002/14651858.CD011020.pub2.PMID: 27364851 Free PMC article. Review.
Source
[ARTICLE] Virtual Reality in the Rehabilitation of Cognitive Impairment after Stroke – Full Text PDF
Posted by Kostas Pantremenos in Cognitive Rehabilitation, Virtual reality rehabilitation on January 7, 2023
Abstract
Virtual reality (VR) is seen by some as a tool that may greatly improve, or even revolutionize cognitive rehabilitation. VR offers distinct advantages compared to classic rehabilitation using paper-and-pencil or computer-based training, such as immersion, the feeling of presence, embodiment of virtual players, ecological and multisensory stimulation. We here review recent clinical studies examining the effects of VR training in patients with stroke-induced cognitive deficits. Several trials reported evidence that VR training improves general cognition compared to standard cognitive training. However, the evidence remains controversial, as some of these studies had a high risk of bias. Regarding mood, there is some indication that immersive training improves depression scores in stroke patients, but the number of studies examining mood changes is very low. Finally, in the domain of spatial cognition the development of specific intervention techniques such as virtual prism adaptation provide avenues for clinical interventions, though well-controlled clinical trials are lacking. Together, the available evidence suggests that VR has the potential to improve rehabilitation particularly in domains requiring repetitive training in an immersed, ecological setting, or when a mismatch between body frames and the environment is created. Controlled clinical studies are required to examine the specific advantages of VR compared to classic interventions.
[BLOG POST] Practice mindfulness to optimize well-being after stroke or brain injury
Posted by Kostas Pantremenos in Cognitive Rehabilitation on December 29, 2022

Practice mindfulness to optimize well-being after stroke or brain injury
by Kate Ying
Mindfulness is a valuable skill that has the ability to enhance your emotional and physical well-being by lowering stress levels and improving cognitive function. These benefits are especially valuable following such a life-changing and challenging event as a stroke or traumatic brain injury.
This post discusses what mindfulness is, how it is beneficial for mental health and cognition, and how to incorporate it into your recovery journey.
What is mindfulness?
Mindfulness has become something of a buzzword recently, but the term actually describes a simple concept. Mindfulness is the state of being fully present and calmly aware of the thoughts, emotions, and sensations you experience from one moment to the next. The goal is to do this curiously and without judgment or attachment to any particular feelings or outcomes.
We all have the potential to be mindful, but certain techniques can help us intentionally activate our inner mindful selves.
Top 4 reasons to practice mindfulness
Studies have shown that becoming more mindful is a simple but powerful way to improve your sense of well-being and sharpen your cognitive abilities. Some specific benefits include:
- Lowered anxiety: Dedicated mindfulness practices can help significantly decrease feelings of anxiety, leading to improved psychological and overall health (Carmody & Baer, 2007).
- Reduced stress: Even a brief mindfulness practice can similarly be an effective intervention to lower psychological stress, which in turn can improve general health. This is especially important if you are recovering from an acute health event, such as a stroke or traumatic brain injury (Creswell et al., 2014).
- Decreased pain and fatigue: Mindfulness practices have been effective in studies at reducing pain and improving mood in survivors of traumatic brain injury (McMillan et al., 2002). Furthermore, survivors of stroke and traumatic brain injury who took a Mindfulness-Based Stress Reduction course reported experiencing decreased mental fatigue (Johansson et al., 2012).
- Improved cognition and communication: Mindfulness practice has also been linked to a longer attention span, which can enhance your ability to perform demanding cognitive tasks and to communicate clearly (Jha et al., 2007). Studies have even shown that consistently meditating might physically alter areas of the brain related to cognitive and sensory processing (Lazar et al., 2005).
How can I practice mindfulness?
One of the best aspects of practicing mindfulness is that it is something anyone can do without special training or equipment. All you need is the desire and willingness to be more fully in touch with the present.
Consider starting here to tap into your inner mindful state:
- Find a quiet moment: Pick a time when you have a few minutes to yourself and will not be rushed or interrupted.
- Commit to practicing for a certain amount of time: It could be a set number of minutes that you track via a timer, or it could simply be for the full duration of a specific activity through to its natural end.
- Start slowly: You might find a mindfulness practice tiring at first, due to the concentration required. It is important to take breaks and go slowly at first, starting with one to two minutes a day and working up.
- Choose your mindfulness exercise: You might try to mindfully taste a sour lemon candy, paying attention to how the wrapper sounds and noting the sensations in your body as the candy melts on your tongue. Or you might decide to mindfully perform a mundane daily activity like washing the dishes, noticing the sound of running water, and the slippery texture of the soap.
Whatever you decide to do, try to do it mindfully. That means that you should try to focus with intention and curiosity on every part of your experience, including what you smell, hear, feel, or think during the practice. Try to avoid fixating on a particular outcome, such as finishing the whole candy or washing all the dishes. Instead, let each moment take you to the next.
- Acknowledge disruptions without judgment or attachment: If you find yourself getting distracted by something external, such as a loud noise, or internal, such as a thought that elicits strong emotion, acknowledge the interruption without judgment of yourself or others. Then, detach yourself from the distraction, gently turning your mind back to the exercise. You might visualize an overwhelming thought or emotion as a leaf floating along a stream, moving toward you and then away again.
- Be patient with yourself: Remember that, as with anything else, the process of becoming more mindful is a gradual one. You might experience impatience, boredom, or frustration as you begin practicing this skill.
Additional supports:
If it feels intimidating to begin a mindfulness practice completely on your own, you might consider the following resources:
- Seek social support: Friends, family, or mental health professionals can be a valuable source of encouragement if you choose to share your mindfulness journey with them. They might remind you to do a daily practice or check-in to ask how you are feeling afterward.
- App-based guided meditations: There are a variety of apps for the iOS and Android operating systems that offer guided mindfulness meditations. Popular apps available for free include Insight Timer, UCLA Mindful, and Smiling Mind.
- Join a local meditation group: Usually, an instructor will lead the group through a live guided meditation. These groups can be a great way to build community into your practice.
- Enroll in a Mindfulness-Based Stress Reduction (MBSR) course: MBSR courses lead students through an intensive, 8-week mindfulness curriculum. They usually involve a substantial time and monetary commitment. Many clinical studies on the benefits of mindfulness have been based on MBSR programs (A.K. and S.K., 2011).
Over time, entering a state of mindfulness will become a more seamless part of your everyday experience. You might continue practicing specific mindfulness techniques or doing daily meditation, or your practice might evolve to integrate more fully with your daily activities. Either way, hopefully, it will not take long before you start to notice the benefits of mindfulness practice on your mental and physical health as you progress in your recovery from a stroke or traumatic brain injury.
References and Further Resources
Creswell, J. D., Pacilio, L. E., Lindsay, E. K., & Brown, K. W. (2014). Brief mindfulness meditation training alters psychological and neuroendocrine responses to social evaluative stress. Psychoneuroendocrinology, 44, 1–12. https://pubmed.ncbi.nlm.nih.gov/24767614/
Carmody, J., & Baer, R. A. (2007). Relationships between mindfulness practice and levels of mindfulness, medical and psychological symptoms and well-being in a mindfulness-based stress reduction program. Journal of Behavioral Medicine, 31(1), 23–33. https://link.springer.com/article/10.1007/s10865-007-9130-7
Jha, A.P., Krompinger, J., & Baime, M.J. (2007). Mindfulness training modifies subsystems of attention. Cognitive, Affective, & Behavioral Neuroscience, 7(2), 109–119. https://link.springer.com/article/10.3758/CABN.7.2.109
Johansson, B., Bjuhr, H., & Rönnbäck, L. (2012). Mindfulness-based stress reduction (MBSR) improves long-term mental fatigue after stroke or traumatic brain injury. Brain Injury, 26(13-14), 1621–1628. https://pubmed.ncbi.nlm.nih.gov/22794665/
Lazar, S. W., Kerr, C. E., Wasserman, R. H., Gray, J. R., Greve, D. N., Treadway, M. T., … Fischl, B. (2005). Meditation experience is associated with increased cortical thickness. NeuroReport, 16(17), 1893–1897. https://pubmed.ncbi.nlm.nih.gov/16272874/
McMillan, T., Robertson, I. H., Brock, D., & Chorlton, L. (2002). Brief mindfulness training for attentional problems after traumatic brain injury: A randomised control treatment trial. Neuropsychological Rehabilitation, 12(2), 117–125. https://www.tandfonline.com/doi/abs/10.1080/09602010143000202
Niazi, A. K., & Niazi, S. K. (2011). Mindfulness-based stress reduction: a non-pharmacological approach for chronic illnesses. North American journal of medical sciences, 3(1), 20–23. https://www.najms.org/article.asp?issn=1947-2714;year=2011;volume=3;issue=1;spage=20;epage=23;aulast=Niazi
Kate Ying is an intern at Constant Therapy Health. She is a student at Columbia University.
[WEB] Memory Motivation
Posted by Kostas Pantremenos in Cognitive Rehabilitation on December 19, 2022

MeMo was designed to respond to the needs of patients with cognitive impairment, of healthcare professionals working on the prevention of cognitive decline, cognitive training and stimulation, as well as of any person willing to train his/her memory and concentration.
MeMo is adapted to patients with the following pathologies:
- Alzheimer’s disease and related disorders
- Traumatic brain injury
- Stroke
- Multiple sclerosis
- Epilepsy
MeMo is the expansion of the work the Innovation Alzheimer association, CoBTeK University of Nice Sophia Antipolis research team working directly with patients from the Nice memory Center, as it provides them with additional ICT-based materials to stimulate and train memory, attention, executive functions, language and gnosis.
MeMo can indeed be employed by clinicians with their patients in individual or group sessions and by the patients alone at home, as a continuation of the work done with the therapist. Till July 2015, MeMo games were regularly proposed to the patients at the Nice Memory Center.

MeMo is freely available on the internet, and can be used on any pc/laptop or tablet.
The exercises are designed and developed by neuropsychologists, medical doctors, researchers and engineers. Every exercise was conceived to train a specific cognitive function, to allow a personalized training based on the observed deficit.
MeMo also allows keeping track of the evolution of performance overtime. After creating an account, the user can track the evolution of his/her performance in the exercises, which can boost motivation and valorize the efforts. This also allows the therapist to follow the evolution of patient’s performance over time.
Our aim is to adapt MeMo and its exercises to the users, by seeking an optimal ease of use. Our team is working continuously to add new games, fix problems and make improvements to the website and the exercises.

Copyright © 2014 – emedinovo