Posts Tagged Virtual rehabilitation
[Abstract] Virtual Rehabilitation Program Using Kinect and Leap Motion Sensor Enhances Upper Limb Function in Stroke Patients
Posted by Kostas Pantremenos in Video Games/Exergames, Virtual reality rehabilitation on January 17, 2023
Abstract:
Virtual reality is being increasingly used in the field of rehabilitation. We tested the benefit of a non-immersive virtual rehabilitation program using the Kinect and Leap Motion sensor in the upper limb rehabilitation in subacute and chronic stroke patients. A total of 30 stroke survivors were randomly assigned in two groups in a 1:1 ratio. The active group benefited from 10 sessions of 30 minutes of virtual rehabilitation program added to the standard rehabilitation therapy. The control group received 10 sessions of 1.5 hours/day of standard rehabilitation therapy during their 14 days hospitalization. Upper limb function was assessed using the Action Research Arm Test (ARAT) and Upper Extremity Fugl-Meyer Assessment (UE-FM) at the beginning and at the end of the 14 days of study. The ARAT and UE-FM improvements were significantly higher in the active group compared to the control group. ARAT improvement was 8.13±5.74 points in the active group versus 2(0.25-2) points in the control group, p=0.0003. The UE-FM improvement was 6.86±3.11 points in the intervention group versus 2.2±1.14 points in the control group, p<0.0001. Virtual rehabilitation therapy was equally effective in the subacute and chronic stroke patients. In the subacute stroke patients, the ARAT improvement was 7.37±6.27 points versus 9±5.41 points in chronic stroke patients (p=0.6). UE-FM improvement in subacute stroke patients was 7.25±3.99 points versus 6.42±1.9 points in chronic patients (p=0.62). Conclusions: Non-immersive virtual reality program improved motor recovery in stroke patients. Both subacute and chronic patients benefited from the virtual therapy.
[ARTICLE] Home-based (virtual) rehabilitation improves motor and cognitive function for stroke patients: a randomized controlled trial of the Elements (EDNA-22) system – Full Text
Posted by Kostas Pantremenos in Cognitive Rehabilitation, Paretic Hand, Tele/Home Rehabilitation, Virtual reality rehabilitation on August 14, 2022
Abstract
Background
Home-based rehabilitation of arm function is a significant gap in service provision for adult stroke. The EDNA-22 tablet is a portable virtual rehabilitation-based system that provides a viable option for home-based rehabilitation using a suite of tailored movement tasks, and performance monitoring via cloud computing data storage. The study reported here aimed to compare use of the EDNA system with an active control (Graded Repetitive Arm Supplementary Program—GRASP training) group using a parallel RCT design.
Methods
Of 19 originally randomized, 17 acute-care patients with upper-extremity dysfunction following unilateral stroke completed training in either the treatment (n = 10) or active control groups (n = 7), each receiving 8-weeks of in-home training involving 30-min sessions scheduled 3–4 times weekly. Performance was assessed across motor, cognitive and functional behaviour in the home. Primary motor measures, collected by a blinded assessor, were the Box and Blocks Task (BBT) and 9-Hole Pegboard Test (9HPT), and for cognition the Montreal Cognitive Assessment (MoCA). Functional behaviour was assessed using the Stroke Impact Scale (SIS) and Neurobehavioural Functioning Inventory (NFI).
Results
One participant from each group withdrew for personal reasons. No adverse events were reported. Results showed a significant and large improvement in performance on the BBT for the more-affected hand in the EDNA training group, only (g = 0.90). There was a mild-to-moderate effect of training on the 9HPT for EDNA (g = 0.55) and control (g = 0.42) groups, again for the more affected hand. In relation to cognition, performance on the MoCA improved for the EDNA group (g = 0.70). Finally, the EDNA group showed moderate (but non-significant) improvement in functional behaviour on the SIS (g = 0.57) and NFI (g = 0.49).
Conclusion
A short course of home-based training using the EDNA-22 system can yield significant gains in motor and cognitive performance, over and above an active control training that also targets upper-limb function. Intriguingly, these changes in performance were corroborated only tentatively in the reports of caregivers. We suggest that future research consider how the implementation of home-based rehabilitation technology can be optimized. We contend that self-administered digitally-enhanced training needs to become part of the health literacy of all stakeholders who are impacted by stroke and other acquired brain injuries.
Trial registration Australian New Zealand Clinical Trials Registry (ANZCTR) Number: ACTRN12619001557123. Registered 12 November 2019, http://www.anzctr.org.au/Trial/Registration/TrialReview.aspx?id=378298&isReview=true
Background
Stroke survivors regard the rehabilitation of upper-limb function as one of the top priorities for increasing their quality of life [1]. However, during the rehabilitation phase the time spent engaged in functional upper-limb activities is often low [2, 3], and at six months after stroke up to 70% remain unable to regain functional use of their affected upper limb(s) [4, 5]. Barriers to therapy include limited access to services, particularly after the transition from acute to in-home care, and low levels of engagement in the rehabilitation program/task itself. These issues have been further compounded by the COVID-19 pandemic, which has highlighted the lack of interventions capable of simultaneously engaging patients in therapy while affording the social distancing and domiciliary options essential for continuity of health care.
Current research shows that optimal recovery from an acquired brain injury (ABI) can be achieved when tailored rehabilitation is provided at high intensity and over a sustained period [7, 8]. Moreover, training tasks should be scaled in complexity (both motor and cognitive) in a manner that accords with the individual needs and capabilities of the patient, fostering motivation and continued progression. To this end, tailored virtual reality (VR), augmented reality (AR) and associated interactive technology can provide a number of key assets for rehabilitation, most notably a medium to increase training doses during critical phases of recovery, scale task difficulty in a systematic way, engage patients’ interest in novel forms of interaction, enhance learning via use of augmented feedback, and record the progress of patients using system-generated metrics. A design principle for many such systems is the notion of enriched therapeutic environments to promote skill acquisition and transfer [9]. The notion here is to present a task environment that not only affords physical movement but also engages the patient’s cognitive attention—both are critical ingredients in skilled performance. This is supported by a recent systematic review and meta-analysis that showed enhanced motor outcomes when these critical ingredients are met with purpose-designed systems [8].
The Elements system (aka EDNA™) [10] was designed originally as a tabletop device (using tangible interfaces) for clinic-based rehabilitation of ABI, with earlier evaluations showing its efficacy for traumatic brain injury [11, 12] and adult stroke [13]. Targeting upper-limb function in TBI patients, significant gains in motor skill were demonstrated in case study [11] and within-groups evaluations [12]. Gains in upper-limb skill also showed positive transfer to everyday function. The most recent RCT extended the application of EDNA to adult stroke and showed strong treatment effects across motor, cognitive and functional outcomes [13]. As well, the experience of using EDNA has been rated highly on the Virtual User Experience Questionnaire (VUE-Q), adapted from the Presence Scale of Witmer and colleagues [14, 15]. Patients have rated highly all six sub-scales: Familiarity, Enjoyment/Engagement, Controllability/Affordance, Efficacy, Social Engagement, and Immersion/Presence, suggesting the system is able to effectively engage the user in the rehabilitation program and promote a sense of improvement. Among the limitations of this in-clinic application, however, is the requirement for one-to-one administration of an adjunct treatment, placing additional demands on the time and resources of rehabilitation services [16].
The capacity to extend access to training into the home environment is particularly important for stroke patients who routinely fail to achieve the recommended doses or durations of therapy necessary to promote meaningful gains [2, 3]. So-called telerehabilitation systems encompass a variety of modalities from videoconferencing, health literacy training delivered over the web, and VR-based systems. Research to date on the benefits of VR- and AR-based therapy in the home are encouraging, but very few controlled trials exist [17]. Evidence suggests, however, that the benefits of such treatment for motor and cognitive function are at least equivalent to standard physical therapy or home-based exercise. In terms of implementation, some guiding principles include the need to “design for engagement” and accommodating the practical challenges of use in the home [18]. These principles speak to the portability of the device and ease of use, providing a viable option for continuity of care as patients transition from the hospital to the home [19].
The EDNA system has therefore recently been extended to include a transportable, tablet device (EDNA-22) for home-based delivery. Targeting upper-limb impairments, the system is designed to provide a viable and flexible therapy option in multiple environments (e.g., clinic, home, and community), which is critical if patients are to achieve recommended doses and durations of rehabilitation [20]. A customised and regular schedule of therapy is delivered via the internet to the patient in their residence, performance data is collected and stored in the cloud, and adherence and performance data is relayed back to the therapist in the clinic. The broad aim of the study presented here was to evaluate the motor, cognitive and functional outcomes of an intensive course of home-based rehabilitation using the EDNA-22 system. First, based on our earlier clinical trials, we expected that participants recovering from stroke would be able to engage effectively in the home-based therapy and adhere to the complete course (i.e., minimum 3 sessions per week, recorded with written log). Second, we expected that the course of therapy would produce significant gains in motor and cognitive function, measured using standardized and validated clinical tools, with the magnitude of changes greater than that observed for an active control therapy (Graded Repetitive Arm Supplementary Program—GRASP) [21, 22]. Third, we expected that patients would also report positive changes in their level of motor functioning. Fourth, we expected that caregivers would report positive change in the general everyday function of patients as a result of the therapy. Finally, we expected the training benefits of EDNA to be maintained across motor, cognitive and functional outcomes at a short-term follow-up (3 months).[…]

[Abstract] Consideration-of-concept of EvolvRehab-Body for upper limb virtual rehabilitation at home for people late after stroke
Posted by Kostas Pantremenos in Paretic Hand, Virtual reality rehabilitation on April 13, 2022
Abstract
Objective
EvolvRehab-Body is a non-immersive virtual rehabilitation system that could provide high-dose, exercise-based upper limb therapy after stroke. This consideration-of-concept study investigated: adherence rate to prescribed repetitions; viability of repeated measures in preparation for a dose-articulation study; and preliminary signal of potential benefit.
Methods
pre-post and repeated measures with people at least six months after stroke. Twelve-week intervention: exercise-based therapy via EvolvRehab-Body. Pre-post-intervention measures: Wolf Motor Function Test (WMFT); hand grip force. Repeated-during-intervention measures: Motricity Index (MI) and Action Research Arm Test (ARAT). Analysis: adherence rate (%) to set repetitions; percentage of total possible measures collected; pre-to-post-intervention change estimated in relation to published minimally detectable changes of WMFT and hand grip force; and slope of plotted data for MI and ARAT (linear regression).
Results
Eight of twelve participants completed the 12-week intervention phase. Adherence: 87.5% (1710 to 9377 repetitions performed). Viability repeated measures: 88 of 96 (91.7%) ARAT and MI scores collected. Preliminary signal of potential benefit was observed in five participants but not always for the same measures. Three participants improved WMFT-time (-7.9 to -27.2 seconds/item), four improved WMFT-function (0.2 to 1.1 points/item), and nobody changed grip force. Slope of plotted data over the 12-week intervention ranged from: -1.42 (p=0.26) to 1.36 (p=0.24) points-per-week for MI and -0.30 (p=0.40) to 1.71 (p<0.001) points-per-week for ARAT.
Conclusion
Findings of good adherence rate in home settings and preliminary signal of benefit for some participants gives support to proceed to a dose-articulation study. These findings cannot inform clinical practice.
[BLOG POST] Futuristic remote tech is empowering a “revolution” in stroke rehab at home
Posted by Kostas Pantremenos in Assistive Technology, REHABILITATION, Tele/Home Rehabilitation, Video Games/Exergames, Virtual reality rehabilitation on November 29, 2021

Thanks to a “technological revolution” in stroke rehabilitation, stroke recoveries could now take a smoother path, with a new interactive health system from Evolv offering telemonitored rehabilitation sessions in stroke survivors’ homes.
Designed with Microsoft’s Azure Kinect camera at its centre, the compact Evolv RehabKit can monitor a user’s precise movements, keeping a close eye on them as they take part in a series of task-based games and exercises. These form part of EvolvRehab, a pioneering virtual rehabilitation platform that runs on the RehabKit.
The therapeutic game-like activities in EvolvRehab have been designed by professional therapists to bolster stroke and brain injury survivors’ self-confidence while increasing their mobility, assisting in their ongoing progression to stand, reach, grasp, walk and speak.Advertisement | Continue story below
Having already seen “life-changing” results in its initial phases, Evolv RehabKit could become even more transformational, according to Microsoft.
Traditional stroke rehabilitation methods are centred around patients having face-to-face sessions in hospitals and specialist centres, which can last for months and sometimes years.
In contrast, the Evolv RehabKit can be used independently at home, with sessions replicating approved exercises, personalised by professional therapists for each survivor. This frees up clinicians’ time and improves patient outcomes through engaging games while easing pressure off the NHS.
David Fried, CEO of Evolv, said: “Our goal was to help deliver rehabilitation out of the clinical setting, providing the repetitive therapeutic activity that’s required to improve outcomes for stroke survivors directly into their homes ideally, or it could be in a day centre, community centre, or residential care home. It can help take the burden off the NHS sites.
“Microsoft is a really big part of that.”
The Evolv RehabKit has also been used to benefit others needing physical rehabilitation, such as those with Parkinson’s, multiple sclerosis, spine and brain injuries, or those who are at risk of falls.
Running from a mini-PC, EvolvRehab is powered by a suite of Microsoft technology, chiefly Azure Kinect, which captures the movement of the user. It also comes with Microsoft Teams, meaning patients and their therapists can communicate via video calls to set goals, share progress and provide feedback.
All the data gathered is stored in the Microsoft Azure cloud. Information and performance results can only be accessed by the user’s authorised physiotherapist. Healthcare professionals can also use Power BI to visualise patient performance and create user reports.
David further highlighted how the Evolv RehabKit offers a service that can’t be replicated elsewhere.
He added: “You need to treat stroke survivors like elite athletes, the same concept, training every day, a healthy lifestyle, positivity and repetition over and over again to improve performance. But you can’t have hundreds of them showing up to an NHS Trust and doing 1000s of repetitions of exercises in a rehabilitation ward.”
The rehabilitation technology has proved particularly helpful during the COVID-19 pandemic, where lockdown restrictions made it impossible for many therapists and stroke patients to meet in person.
In September 2020, Microsoft helped Evolv as it made more RehabKits available to healthcare teams. These were given to stroke survivors, allowing their vital progress to continue at home.
“What we try to do in our platform is offer different types of activities that touch upon different types of therapy. The important thing is the activities can be personalised to each individual at home,” David continued.
“For example, you’d be playing a game where barriers come out towards you, and you have to lift your leg to get over them, which helps balance and coordination. They are games, you are doing repetition, you are doing therapeutic activity that’s prescribed and personalised to you, but you have no idea you are doing exercise – you are simply playing a game.”
Evolv works closely with the specialist National Hospital for Neurology and Neurosurgery in Queen Square, London, which is part of University College London Hospitals NHS Foundation Trust. It has also partnered with academic institutions such as the universities of Reading, East Anglia and Cambridge, as well as collaborating with private physiotherapy providers.
While its physical challenges offer upper and lower extremity training, and fine motor skills for the hand, EvolvRehab also contains dual-tasking ‘exergames’ (exercise while playing gaming). These combine language and motor training to encourage thinking and moving simultaneously.
For example, a word is displayed on-screen along with several pictures of different items, and the “player” must reach out and touch the correct picture of the item based on the given word. At other times, they will touch the item that rhymes with a given word or starts with a certain sound.
It is through gamifying physiotherapy with points and trophies that the EvolvRehab software ensures survivors want to continue treatment, according to Microsoft.
Evolv is part of the Microsoft for Startups and Global Social Entrepreneurship programme, a global programme that offers new companies access to technology and go-to-market and community benefits to help them grow.
EvolvRehab is currently available in 10 different languages, with clinical validation in seven countries, and has been used to provide telerehabilitation in a dozen countries.
[Abstract + References] Enhancing mirror therapy via scaling and shared control: a novel open-source virtual reality platform for stroke rehabilitation
Posted by Kostas Pantremenos in Mirror therapy, Virtual reality rehabilitation on October 19, 2021
Abstract
Mirror therapy is increasingly used in stroke rehabilitation to improve functional movements of the affected limb. However, the extent of mirroring in conventional mirror therapy is typically fixed (1:1) and cannot be tailored based on the patient’s impairment level. Further, the movements of the affected limb are not actively incorporated in the therapeutic process.
To address these issues, we developed an immersive VR system using HTC Vive and Leap Motion, which communicates with our free and open-source software environment programmed using SteamVR and the Unity 3D gaming engine. The mirror therapy VR environment was incorporated with two novel features: (1) scalable mirroring and (2) shared control. In the scalable mirroring, mirror movements were programmed to be scalable between 0 and 1, where 0 represents no movements, 0.5 represents 50% mirroring, and 1 represents 100% mirroring. In shared control, the contribution of the mirroring limb to the movements was programmed to be scalable between 0 to 1, where 0 represents 100% contribution from the mirroring limb (i.e., no mirroring), 0.5 represents 50% of movements from the mirrored limb and 50% of movements from the mirroring limb, and 1 represents full mirroring (i.e., no shared movements).
Validation experiments showed that these features worked appropriately. The proposed VR-based mirror therapy is the first fully developed system that is freely available to the rehabilitation science community. The scalable and shared control features can diversify mirror therapy and potentially augment the outcomes of rehabilitation, although this needs to be verified through future experiments.
References
- Abbink DA, Mulder M, Boer ER (2012) Haptic shared control: smoothly shifting control authority? Cogn Technol Work 14:19–28. https://doi.org/10.1007/s10111-011-0192-5Article Google Scholar
- Arya KN, Pandian S (2013) Effect of task-based mirror therapy on motor recovery of the upper extremity in chronic stroke patients: a pilot study. Top Stroke Rehabil 20:210–217. https://doi.org/10.1310/tscir2001-210Article Google Scholar
- Arya KN, Pandian S, Kumar D, Puri V (2015) Task-based mirror therapy augmenting motor recovery in poststroke hemiparesis: a randomized controlled trial. J Stroke Cerebrovasc Dis 24:1738–1748. https://doi.org/10.1016/j.jstrokecerebrovasdis.2015.03.026Article Google Scholar
- Arya KN, Pandian S, Kumar V (2019) Effect of activity-based mirror therapy on lower limb motor-recovery and gait in stroke: a randomised controlled trial. Neuropsychol Rehabil 29:1193–1210. https://doi.org/10.1080/09602011.2017.1377087Article Google Scholar
- Bai Z, Fong KNK, Zhang J, Hu Z (2020) Cortical mapping of mirror visual feedback training for unilateral upper extremity: a functional near-infrared spectroscopy study. Brain Behav. https://doi.org/10.1002/brb3.1489Article Google Scholar
- Broderick P, Horgan F, Blake C et al (2018) Mirror therapy for improving lower limb motor function and mobility after stroke: a systematic review and meta-analysis. Gait Posture 63:208–220. https://doi.org/10.1016/j.gaitpost.2018.05.017Article Google Scholar
- Crosbie JH, Lennon S, Basford JR, McDonough SM (2007) Virtual reality in stroke rehabilitation: still more virtual than real. Disabil Rehabil 29:1139–1146. https://doi.org/10.1080/09638280600960909Article Google Scholar
- Fritzsch C, Wang J, Dos Santos LF et al (2014) Different effects of the mirror illusion on motor and somatosensory processing. Restor Neurol Neurosci 32:269–280. https://doi.org/10.3233/RNN-130343Article Google Scholar
- Garry MI, Loftus A, Summers JJ (2005) Mirror, mirror on the wall: viewing a mirror reflection of unilateral hand movements facilitates ipsilateral M1 excitability. Exp Brain Res 163:118–122. https://doi.org/10.1007/s00221-005-2226-9Article Google Scholar
- González-Franco M, Pérez-Marcos D, Spanlang B, Slater M (2010) The contribution of real-time mirror reflections of motor actions on virtual body ownership in an immersive virtual environment. Proc IEEE Virtual Real. https://doi.org/10.1109/VR.2010.5444805Article Google Scholar
- Heinrich C, Cook M, Langlotz T, Regenbrecht H (2020) My hands? Importance of personalised virtual hands in a neurorehabilitation scenario. Virtual Real. https://doi.org/10.1007/s10055-020-00456-4Article Google Scholar
- Hoermann S, Ferreira Dos Santos L, Morkisch N et al (2017) Computerised mirror therapy with augmented reflection technology for early stroke rehabilitation: clinical feasibility and integration as an adjunct therapy. Disabil Rehabil 39(15):1503–1514. https://doi.org/10.1080/09638288.2017.1291765Article Google Scholar
- In T, Lee K, Song C (2016) Virtual reality reflection therapy improves balance and gait in patients with chronic stroke: randomized controlled trials. Med Sci Monit 22:4046–4053. https://doi.org/10.12659/msm.898157Article Google Scholar
- Kang YJ, Park HK, Kim HJ et al (2012) Upper extremity rehabilitation of stroke: facilitation of corticospinal excitability using virtual mirror paradigm. J Neuroeng Rehabil. https://doi.org/10.1186/1743-0003-9-71Article Google Scholar
- Kim K, Lee S, Kim D et al (2016) Effects of mirror therapy combined with motor tasks on upper extremity function and activities daily living of stroke patients. J Phys Ther Sci 28:483–487. https://doi.org/10.1589/jpts.28.483Article Google Scholar
- Kleim JA, Jones TA (2008) Principles of experience-dependent neural plasticity: Implications for rehabilitation after brain damage. J Speech Lang Hear Res 51
- Lee D, Lee M, Lee K, Song C (2014) Asymmetric training using virtual reality reflection equipment and the enhancement of upper limb function in stroke patients: a randomized controlled trial. J Stroke Cerebrovasc Dis 23:1319–1326. https://doi.org/10.1016/j.jstrokecerebrovasdis.2013.11.006Article Google Scholar
- Lu EC, Wang RH, Hebert D et al (2011) The development of an upper limb stroke rehabilitation robot: identification of clinical practices and design requirements through a survey of therapists. Disabil Rehabil Assist Technol 6:420–431. https://doi.org/10.3109/17483107.2010.544370Article Google Scholar
- Morales-Rodriguez ML, Pavard B (2007) Design of an emotional and social interaction paradigm for the animation of 3D characters: the case of a therapy for brain injured people (the mirror neuron paradigm). Virtual Real 11:175–184. https://doi.org/10.1007/s10055-006-0063-1Article Google Scholar
- Morkisch N, Thieme H, Dohle C (2019) How to perform mirror therapy after stroke? Evidence from a meta-analysis. Restor Neurol Neurosci 37:421–435. https://doi.org/10.3233/RNN-190935Article Google Scholar
- O’Sullivan N, de Bezenac C, Piovesan A et al (2018) I Am There … but Not Quite: an unfaithful mirror that reduces feelings of ownership and agency. Perception 47:197–215. https://doi.org/10.1177/0301006617743392Article Google Scholar
- Organization WH (2002) The world health report 2002: reducing risks, promoting healthy life
- Park Y, Chang M, Kim K-M, An D-H (2015) The effects of mirror therapy with tasks on upper extremity function and self-care in stroke patients. J Phys Ther Sci 27:1499–1501. https://doi.org/10.1589/jpts.27.1499Article Google Scholar
- Ramachandran VS, Rogers-Ramachandran D (2019) Mirror feedback assisted recovery from hemiparesis following stroke. in Reply to Morkisch et al.: How to perform mirror therapy after stroke? Evidence from a meta-analysis. Restor Neurol Neurosci 37:437–443Google Scholar
- Ramachandran VS, Rogers-Ramachandran D, Cobb S (1995) Touching the phantom limb. Nature 377:489–490. https://doi.org/10.1038/377489a0Article Google Scholar
- Ranganathan R (2017) Reorganization of finger coordination patterns through motor exploration in individuals after stroke. J Neuroeng Rehabil. https://doi.org/10.1186/s12984-017-0300-8Article Google Scholar
- Samuelkamaleshkumar S, Reethajanetsureka S, Pauljebaraj P et al (2014) Mirror therapy enhances motor performance in the paretic upper limb after stroke: a pilot randomized controlled trial. Arch Phys Med Rehabil 95:2000–2005. https://doi.org/10.1016/j.apmr.2014.06.020Article Google Scholar
- Sanchez-Vives MV, Slater M (2005) From presence to consciousness through virtual reality. Nat Rev Neurosci 6:332–339Article Google Scholar
- Subramanian SK, Levin MF (2011) Viewing medium affects arm motor performance in 3D virtual environments. J Neuroeng Rehabil. https://doi.org/10.1186/1743-0003-8-36Article Google Scholar
- Subramanian SK, Lourenço CB, Chilingaryan G et al (2013) Arm motor recovery using a virtual reality intervention in chronic stroke: randomized control trial. Neurorehabil Neural Repair 27:13–23. https://doi.org/10.1177/1545968312449695Article Google Scholar
- Subramanian SK, Cross MK, Hirschhauser CS (2020) Virtual reality interventions to enhance upper limb motor improvement after a stroke: commonly used types of platform and outcomes. Disabil Rehabil Assist Technol
- Thieme H, Morkisch N, Mehrholz J, et al (2018) Mirror therapy for improving motor function after stroke (Review) SUMMARY OF FINDINGS FOR THE MAIN COMPARIS1. Kawakami K, Miyasaka H, Nonoyama S, et al. Randomized controlled comparative study on effect of training to improve lower limb motor paralysis. Cochrane Database Syst Rev 1–182. doi:https://doi.org/10.1002/14651858.CD008449.pub3.www.cochranelibrary.com
- Virani SS, Alonso A, Benjamin EJ et al (2020) Heart disease and stroke statistics—2020 update: a report from the American Heart Association. Circulation 141:E139–E596Google Scholar
- Weber LM, Nilsen DM, Gillen G et al (2019) Immersive virtual reality mirror therapy for upper limb recovery after stroke: a pilot study. Am J Phys Med Rehabil 98:783–788. https://doi.org/10.1097/PHM.0000000000001190Article Google Scholar
- Zhu M-H, Zeng M, Shi M-F et al (2020) Visual feedback therapy for restoration of upper limb function of stroke patients. Int J Nurs Sci 7:170–178. https://doi.org/10.1016/j.ijnss.2020.04.004Article Google Scholar
[BLOG POST] Telerehabilitation – CNS Traumatic Brain Injury Rehabilitation
Posted by Kostas Pantremenos in REHABILITATION, Tele/Home Rehabilitation on October 15, 2021

TELEREHABILITATION
INSTANT ACCESS TO THERAPIES AND PHYSICIANS. ANYTIME, ANYWHERE
Since 2020, CNS has delivered more than 125,000 hours of virtual rehabilitation to patients.
Telerehab offers patients greater flexibility and is a valuable stage in the patient’s continuum of care. Using the RingCentral Video® platform, powered by Zoom, patients can now access therapies, clinicians, and their treatment team with ease.
Services Include
- Telerehab: Patients receive full rehabilitation at home, virtually, from their therapy team working at a CNS clinic.
- Telerehab Kit: CNS ships a boxed kit of learning aids to telerehab and some hybrid patients. Included is a user’s guide for navigating RingCentral Video, learning tools, notebooks, calculator, pens, a water bottle and a desktop whiteboard.
- Hybrid Rehabilitation: If appropriate, patients can opt for both in-clinic (in person) and virtual (in home) treatment.
- Telemedicine: Patients, therapists and families can consult online with physicians as needed.
Telerehab Systems and Features
Seamless Collaboration with RingCentral Video and Zoom
Privacy and patient safety are critical to delivering patient care virtually. The RingCentral Video solution and Zoom are secure, HIPAA compliant platforms deployed in all CNS clinics. This enables patients and therapy teams to connect, communicate, and collaborate seamlessly. Integrated video meetings and messaging also allow anyone in the patient’s sphere to communicate.
Therapy in Real Time: Productive, Interactive, Effective
- All treatment modules are provided; vital living skills are taught
- In-clinic therapies are replicated in the patient’s home setting
- Staff can suggest adaptive aids by viewing the home environment
- Return to work scenarios can be modeled and practiced at home
- Therapists teach safety skills; patients can practice in real time
Virtual Collaboration: Family/Clinician Meetings, Simplified
- Staff will arrange online conferences with MDs, patients, family
- Therapy teams collaborate to modify treatment plans
- Evaluation and admissions processes can be initiated
- Discharge planning can be discussed with patient’s sphere
- Therapists provide family guidance for challenging issues
Physician Consultations: Professional, Private, Immediate
Doctors examine, diagnose, suggest treatment for:
- Physical challenges
- Behavioral concerns
- Medical management and comorbidity care
- Post COVID-19 recovery
Telemedicine also reduces the need for urgent care and emergency room visits, as certain evaluations and physician input can be accomplished via video and sharing patient data.
CNS TELEREHAB PROGRAM KIT
[Abstract+References] Fatigue-aware videogame using biocybernetic adaptation: a pilot study for upper-limb rehabilitation with sEMG
Posted by Kostas Pantremenos in Paretic Hand, REHABILITATION, Video Games/Exergames on August 3, 2021
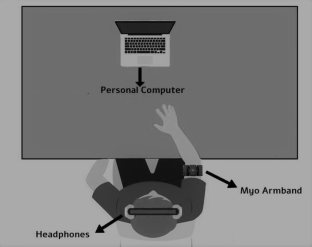
Abstract
Physical rehabilitation has been widely used to restore or maintain motor capabilities of patients with upper-limb mobility limitations. Despite its effectiveness, physical rehabilitation has several difficulties in engaging patients with the multiple therapeutical sessions required to obtain measurable benefits. Novel technologies incorporate gamification strategies to encourage participants to play during the rehabilitation sessions (instead of counting repetitions), providing benefits for therapy adherence. “Serious” or also called applied games have been used as a complementary therapy for neuromuscular disorders. However, the therapy effectiveness of several serious games for health has been questioned by the clinical experts since crucial factors associated with the physical rehabilitation are not commonly included in the gameplay. This study reports the use of a physiologically aware serious game developed using surface electromyography (sEMG) to capture upper-limb muscular fatigue levels of participants. We carried out a pilot study lasting four weeks with five participants diagnosed with monoparesis/hemiparesis to evaluate the feasibility of using the fatigue-adaptive game called Force Defense as a complementary tool for physical rehabilitation in a local community-based rehabilitation center. Preliminary results suggest a positive user gameplay experience as well as good usability of the system reported by participants after the first intervention session. Moreover, we showed how the physiological adaptation was able to encourage participants to maintain exertion in the therapeutically desired zone, thus improving the system’s effectiveness. Participants also improved in their functional abilities of the upper limbs and the game performance measured in pre- and post-moments and reported reduced levels of perceived fatigue after the end of the training program.
References
- Agredo CA, Bedoya JM (2005) Validación de la escala ashworth modificada. Arq Neuropsiquiatr 3:847–851Google Scholar
- Bonnechère B (2018) Serious games in physical rehabilitation. Springer. https://doi.org/10.1007/978-3-319-66122-3Article Google Scholar
- Borg, G. (1998). Borg’s perceived exertion and pain scales. Human kinetics.
- Brooke J (1996) SUS-A quick and dirty usability scale. Usability Evaluation in Industry 189(194):4–7Google Scholar
- Brooks AL (2018) Recent Advances in Technologies of Inclusive Well-Being: Virtual patients, gamification and simulation: Springer series Intelligent Systems Reference Library Indexed by DBLP. MetaPress and Springerlink, Ulrichs, SCOPUS, MathSciNet, Current Mathematical Publications, Mathematical Reviews, Zentralblatt Math. https://doi.org/10.1007/978-3-319-49879-9Book Google Scholar
- Borg E, Borg G, Larsson K, Letzter M, Sundblad BM (2010) An index for breathlessness and leg fatigue. Scand J Med Sci Sports 20(4):644–650. https://doi.org/10.1111/j.1600-0838.2009.00985.xArticle Google Scholar
- Collange Grecco LA, de Almeida Carvalho Duarte N, Mendonça ME, Galli M, Fregni F, Oliveira CS (2015) Effects of anodal transcranial direct current stimulation combined with virtual reality for improving gait in children with spastic diparetic cerebral palsy: a pilot, randomized, controlled, double-blind, clinical trial. Clin Rehabil 29(12):1212–1223. https://doi.org/10.1177/0269215514566997 Article Google Scholar
- Corbetta D, Imeri F, Gatti R (2015) Rehabilitation that incorporates virtual reality is more effective than standard rehabilitation for improving walking speed, balance and mobility after stroke: a systematic review. J Physiother 61(3):117–124. https://doi.org/10.1016/j.jphys.2015.05.017Article Google Scholar
- Cram JR (2011) Cram’s introduction to surface electromyography. Jones & Bartlett Learning, BurlingtonGoogle Scholar
- Csikszentmihalyi M (2000) Beyond boredom and anxiety. Jossey-BassGoogle Scholar
- De Luca CJ (1997) The use of surface electromyography in biomechanics. J Appl Biomech 13(2):135-163. https://doi.org/10.1123/jab.13.2.135Article Google Scholar
- Dörner R, Göbel S, Kickmeier-Rust M, Masuch M, Zweig K (2016) Entertainment Computing and Serious Games: International GI-Dagstuhl Seminar 15283, Dagstuhl Castle, Germany, July 5-10, 2015, Revised Selected Papers (Vol. 9970). Springer. https://doi.org/10.1007/978-3-319-46152-6
- Fairclough S, Gilleade K (2012) Construction of the biocybernetic loop: a case study. In: Proceedings of the 14th ACM international conference on Multimodal interaction, pp. 571–578. https://doi.org/10.1145/2388676.2388797
- Fairclough SH, Gilleade K (2014) Advances in physiological computing. Springer, LondonBook Google Scholar
- Galiano-Castillo N, Ariza-García A, Cantarero-Villanueva I, Fernández-Lao C, Díaz-Rodríguez L, Arroyo-Morales M (2014) Depressed mood in breast cancer survivors: Associations with physical activity, cancer-related fatigue, quality of life, and fitness level. Eur J Oncol Nurs 18(2):206–210. https://doi.org/10.1016/j.ejon.2013.10.008Article Google Scholar
- Hocine N, Gouaich A, Di Loreto I, Joab M (2011) Motivation based difficulty adaptation for therapeutic games. In: 2011 IEEE 1st International Conference on serious games and applications for health (SeGAH), pp. 1–8. DOI: https://doi.org/10.1109/SeGAH.2011.6165459
- IJsselsteijn WA, De Kort YAW, Poels K (2008) The game experience questionnaire. Manuscript in preparation.
- Jayaram S, Connacher HI, Lyons KW (1997) Virtual assembly using virtual reality techniques. Comput Aided Des 29(8):575–584. https://doi.org/10.1016/S0010-4485(96)00094-2Article Google Scholar
- Kourtis LC, Regele OB, Wright JM, Jones GB (2019) Digital biomarkers for Alzheimer’s disease: the mobile/wearable devices opportunity. NPJ Digit Med 2(1):1–9. https://doi.org/10.1038/s41746-019-0084-2Article Google Scholar
- Laver KE, Lange B, George S, Deutsch JE, Saposnik G, Crotty M (2017) Virtual reality for stroke rehabilitation. Cochrane Datab Syst Rev. https://doi.org/10.1002/14651858.CD008349.pub4Article Google Scholar
- Levac DE, Sveistrup H (2014) Motor learning and virtual reality. Virtual reality for physical and motor rehabilitation. Springer, Cham, pp 25–46Google Scholar
- Manera V, Ben-Sadoun G, Aalbers T, Agopyan H, Askenazy F, Benoit M, Bensamoun D, Bourgeois J, Bredin J, Bremond F (2017) Recommendations for the use of serious games in neurodegenerative disorders: 2016 Delphi Panel. Front Psychol 8:1243. https://doi.org/10.3389/fpsyg.2017.01243Article Google Scholar
- Merletti R (2000) Surface electromyography: The SENIAM project. Eur J Phys Rehabil Med 36(4):167Google Scholar
- Merletti R, Farina D (2016) Surface electromyography: physiology, engineering and applications. John Wiley & Sons, New YorkBook Google Scholar
- Montoya M, Henao O, Muñoz J (2017) Muscle fatigue detection through wearable sensors: a comparative study using the myo armband. Proc XVIII Int Conf Human Comput Interact. https://doi.org/10.1145/3123818.3123855Article Google Scholar
- Montoya MF, Muñoz J, Henao O (2019) Design of an upper limbs rehabilitation videogame with sEMG and biocybernetic adaptation. In: Proceedings of the 5th Workshop on ICTs for improving Patients Rehabilitation Research Techniques, pp. 152–155. https://doi.org/10.1145/3364138.3364170
- Montoya MF, Muñoz JE, Henao OA (2020) Enhancing virtual rehabilitation in upper limbs with biocybernetic adaptation: the effects of virtual reality on perceived muscle fatigue, game performance and user experience. IEEE Trans Neural Syst Rehabil Eng 28(3):740–747. https://doi.org/10.1109/TNSRE.2020.2968869Article Google Scholar
- Muñoz JE, Cameirão M, Bermúdez Badia S, Gouveia ER (2018) Closing the loop in exergaming-health benefits of biocybernetic adaptation in senior adults. In: Proceedings of the 2018 Annual Symposium on Computer-Human Interaction in Play, pp. 329–339. https://doi.org/10.1145/3242671.3242673
- Newbutt N (2015) Technologies of inclusive well-being: serious games, alternative realities, and play therapy. EMERALD GROUP PUBLISHING LTD HOWARD HOUSE, WAGON LANEGoogle Scholar
- Pons JL, Torricelli D (2014) Emerging therapies in neurorehabilitation. Springer, ChamBook Google Scholar
- Puddu G, Giombini A, Selvanetti A (2013) Rehabilitation of sports injuries: current concepts. Springer, ChamGoogle Scholar
- Rawat S, Vats S, Kumar P (2016) Evaluating and exploring the MYO ARMBAND. Int Conf Syst Model Adv Res Trends (SMART) 2016:115–120. https://doi.org/10.1109/SYSMART.2016.7894501Article Google Scholar
- Sawyer B (2008) From cells to cell processors: the integration of health and video games. IEEE Comput Graphics Appl 28(6):83–85. https://doi.org/10.1109/MCG.2008.114Article Google Scholar
- Serbedzija NB, Fairclough SH (2009) Biocybernetic loop: from awareness to evolution. IEEE Congr Evol Comput 2009:2063–2069. https://doi.org/10.1109/CEC.2009.4983195Article Google Scholar
- Sinclair J, Hingston P, Masek M (2009) Exergame development using the dual flow model. Proc Sixth Austral Conf Interact Entertain. https://doi.org/10.1145/1746050.1746061Article Google Scholar
- Taub E, Uswatte G, Mark VW, Morris DM, Barman J, Bowman MH, Bryson C, Delgado A, Bishop-McKay S (2013) Method for enhancing real-world use of a more affected arm in chronic stroke: transfer package of constraint-induced movement therapy. Stroke 44(5):1383–1388. https://doi.org/10.1161/STROKEAHA.111.000559Article Google Scholar
[Infographic] Classification of sensor types used in virtual rehabilitation for upper limb rehabilitation
Posted by Kostas Pantremenos in Paretic Hand, Virtual reality rehabilitation on October 27, 2020
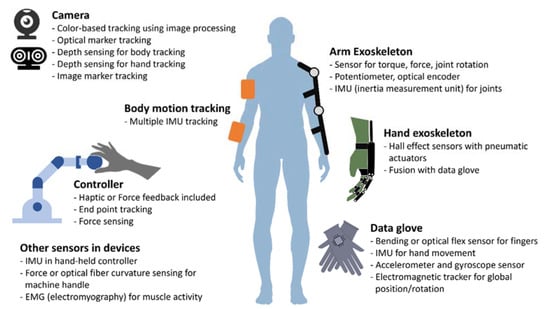
[WEB PAGE] Virtual Rehabilitation Programs for Stroke have many Benefits, says Study!!!
Posted by Kostas Pantremenos in REHABILITATION, Tele/Home Rehabilitation, Virtual reality rehabilitation on September 7, 2020
By Nilanjana Roy

A study shows the benefits of virtual stroke Of rehabilitation. In a recent review paper by Brodie Sakakibara with the center for chronic disease prevention and management has determined that virtual appointment in the form of telerehabilitation also works for people recovering from a stroke.
After a stroke a client provided with a therapy program to regain the loss of skills or motion this can range from speech and memory, strength, balance, and endurance. Sakakibara a UBCO assistant professor says research shows remote therapy can be effective during stroke recovery. Telerehabilitation has promoted as a more efficient means of delivering rehabilitation services to stroke patients while also providing care options to those who attend conventional therapy.
These services can be provided to remote locations through information and communication technology and can be accessed by the patients from their homes. To learn effective telerehabilitation six different clinical trials have been launched across Canada as a part of the heart and stroke foundation initiated. People who recovered from strokes were given intervention ranging from lifestyle vouching to. Memory speech skills and physical exercise training.
Research for every six trials came together to write a review paper to describe the experience of conducting a telerehabilitation study. And reports on the facilitators and barriers to implementing the telerehabilitation services.
Going with telerehabilitation As a new reality, the study determines there are important lessons to learn from. Each of the six trials. Most importantly the efficiency and cost of telerehabilitation are similar to the face to face management. Patients have mostly reported satisfaction from the telerehabilitation when therapists have trained appropriately and with some social interaction. Clinicians prefer face to face interaction but will use telerehabilitation when face to face is not feasible.
[Conference Paper] HoVRS: Home-based Virtual Rehabilitation System – Full Text
Posted by Kostas Pantremenos in Paretic Hand, REHABILITATION, Tele/Home Rehabilitation, Virtual reality rehabilitation on March 8, 2020
ABSTRACT
After stroke, sustained hand rehabilitation training is required for continuous improvement and maintenance of distal function. In this paper, we present a system designed and implemented in our lab: the Home-based Virtual Rehabilitation System (HoVRS). Eleven subjects with chronic stroke were recruited to test the feasibility of the system and refine its design and the training protocol to prepare for a future efficacy study. HoVRS was placed at subjects’ homes, and subjects were asked to use the system at least 15 minutes every weekday for 3 months (12 weeks) with limited technical support and remote clinical monitoring. All subjects completed the study without any adverse events. Subjects on average spent 12 hours using the system. Nine out of the eleven subjects improved on the Box and Blocks Test (BBT), and ten improved on the Upper Extremity Fugl-Meyer Assessment (FM) and the Action Research Arm Test (ARAT). The outcomes of this pilot study warrant further investigation of the system’s ability to promote recovery of hand function in subacute and chronic stroke.
INTRODUCTION
Stroke is a leading cause of serious long-term disability in the United States. The incidence of new or recurrent stroke in the US is 795,000 per year and the prevalence of chronic stroke is approximately 7 million (Go et al., 2014). Projections show that by 2030, an additional 3.4 million people or 3.88% of U.S. adults 18 and older will have had a stroke, a 20.5% increase from 2012 (American Stroke Association 2018). At six months post-stroke, about 65% of affected persons continue to have hand deficits that profoundly affect their ability to perform their usual activities and their independence (Dobkin, 2005; Lang, et al. 2006). This leaves a potential market segment of approximately 640,000 persons that may need long-term arm and hand rehabilitation. Restoration of hand function is of utmost importance since it is the loss of hand function that profoundly decreases quality of life by limiting the ability to perform feeding, dressing, and grooming, and further may limit the use of assistive as well as telecommunications technology (Brown et al. 1987, Grimby et al. 1998, Andren et al. 2004, Kwakkel et al. 2008).
Therapy in an inpatient rehabilitation center only lasts about 2-3 weeks. As outpatients, stroke survivors are typically only seen two to three times a week for short time periods. This volume of intervention falls far short of the hundreds of hours needed to re-establish normal hand function. Recently published results of innovative lab-based interventions appear to have a similar problem (Lang et al., 2015, Winstein et al., 2016). It is therefore imperative to develop an intervention that can be delivered at home over a sufficient period of time to elicit improvements.
Innovative telerehabilitation systems have been developed using information and communication technologies to provide rehabilitation services at a distance. Many studies have developed video-game driven systems from commercially available gaming consoles such as Wii and Microsoft Kinect (Metcalf et. al, 2013), however, these systems do not address hand rehabilitation. Other groups, including members of our own team, have examined the use of custom-made telerehabilitation systems (Adamovich et. al, 2005, Turolla et. al., 2013) but they are not commercially available. An ideal home-based telerehabilitation system has to be low cost, easy to setup, able to motivate the user for everyday use, generate progress reports for the user for self-tracking, and provide daily monitoring to remote clinicians. Exciting new technologies have now made this approach possible and hold promise for long-term benefit. These technological advances – for the first time – allow for virtual reality simulations interfaced with discrete finger and hand tracking that are affordable and easy to use.
Our product, the Home Virtual Rehabilitation System (HoVRS), provides intense upper extremity rehabilitation at home. It will allow patients to access hand/arm rehabilitation without the cost and transportation challenges associated with outpatient rehabilitation. HoVRS will consist of five elements: 1) an infrared camera specifically designed to capture finger and arm movements – a substantial improvement over rehabilitation activities provided by commercial game consoles like Kinect or Wii, 2) multiple engaging games that train the hand and arm using commercial gaming mechanics designed to optimize players’ motivation to perform these activities for long periods of time, 3) an optional exoskeleton designed to assist the patient’s arm as it moves against gravity (use of this support can be weaned and eliminated as patients get stronger), 4) monitoring and archiving software that will allow clinicians to design custom rehabilitation interventions, track a patient’s progress, and modify a patient’s rehabilitation program, in-person or remotely, and 5) a secure wireless data connector to collect detailed information on patient movement in real time. The secure communication channel will allow for remote monitoring by clinicians, remote technical support, and remote patient and clinician interaction face to face, while the patient uses HoVRS.
This study describes the experiences of the first eleven persons with stroke that participated in pilot testing of HoVRS in their homes.[…]
Continue —-> HoVRS: Home-based Virtual Rehabilitation System

Figure 1. HoVRS architecture design